Impact of leg heating on central hemodynamics in postmenopausal women
- DOI
- 10.1016/j.artres.2018.01.001How to use a DOI?
- Keywords
- Central pressure augmentation; Wave reflection; Pulse wave velocity; Central-to-leg arterial stiffness gradient
- Abstract
Background: The determinants responsible for increasing central pressure augmentation, a strong risk factor for cardiovascular disease, remain highly controversial. The aim of this study was to determine the contribution of the impedance mismatch between central and leg arteries on central pressure augmentation. Thus, we investigated whether central pressure augmentation is influenced by manipulation of central-to-leg arterial stiffness mismatch by an acute leg heating.
Methods: Nineteen postmenopausal women underwent the warmth stimulation on both lower legs (20 min of exposure to far infra-red radiation at 43–45 °C followed by retaining warmth by a blanket for 30 min). Central (aortic) hemodynamic measures were obtained from applanation tonometrically-radial arterial pressure waveforms via the validated general transfer function.
Results: The leg heating decreased only the leg pulse wave velocity. And thus, the central-to-leg arterial stiffness was changed from positive to negative; however, any central (aortic) hemodynamic measures including reflected wave amplitude, aortic blood pressure, and augmentation index, were not changed significantly.
Conclusions: Our results suggest that the acute bout of leg heating induces the less of central-to-leg arterial stiffness mismatch but not influence central pressure augmentation in postmenopausal women.
- Copyright
- © 2018 Association for Research into Arterial Structure and Physiology. Published by Elsevier B.V. All rights reserved.
- Open Access
- This is an open access article distributed under the CC BY-NC license.
Introduction
Enhanced central pressure augmentation, evaluated by augmented pressure (AP) and augmentation index (AIx), could be a strong risk for cardiovascular disease (CVD).1 Postmenopausal women exhibit greater central pressure augmentation compared with premenopausal women and age-matched men.2
There is a widely held notion that the central arterial pressure waveform is synthesized by the overlapped reflection waves returning from the periphery (mainly lower body) on the incident wave in phase.3 AP and AIx are associated with the extent and timing of reflected wave that arises from the periphery.3 In the arterial tree, a traveling wave will be reflected to some extent wherever there is a discontinuity such as branching points (e.g., branches of renal arteries, aortic bifurcations), areas of alteration in arterial stiffness (e.g., from the elastic artery to the muscular artery), and high-resistance arterioles.3 Therefore, alterations of peripheral vascular tone and arterial stiffness may influence central pressure augmentation.
Recently, however, this theory has been argued. Growing evidences suggest that major determinants of central BP waveform may be the incident waves arising from left ventricular ejection and proximal aortic compliance rather than the wave reflection.4–10 For example, a community-based cross-sectional investigation revealed that aortic-brachial artery stiffness mismatch was not associated with the central AP and AIx.11 An interventional experiment supported this result that the central hemodynamics were unaltered even if the brachial vascular tone was changed using an arm heating.12 On the other hand, it remains unclear whether the impedance mismatch between central and leg arteries influences central pressure augmentation.
From ancient times, a leg thermal therapy with comfortable temperature (≈40 °C) such as “footbath” has been widely used for the purpose of improving the whole-body hemodynamics and keeping warm. In particular, the lower leg heating has an advantage that it can be carried out everyone easily and safely without the need for undressing. It is well known that the warmth stimulation elicits local various vasodilatory substances typified by nitric oxide.13,14 In fact, it has been reported that an isolated leg heat stress increases leg blood flow and vascular conductance.15 They may induce not only dilatation in local (lower leg) vascular bed but also upstream hyperemia (e.g., femoral arterial blood flow) and consequent dilation of conduit arteries because of the increases in downstream vascular conductance and inflow. If it is so, leg arterial stiffness could be decreased, and hence, arterial stiffness mismatch between central and leg arteries is attenuated. Taken together, the lower leg heating may change wave reflection and central pressure augmentation via decreased vascular resistance and less arterial stiffness mismatch even though acutely.
With this information as background, in the present study, we determined the effect of an acute bout of lower leg heating on central pressure augmentation in postmenopausal women.
Methods
Subjects
We studied 19 postmenopausal women (age, 64 ± 3 years; height, 154 ± 6 cm; weight, 53 ± 7 kg; mean ± SD). All the subjects had no apparent cardiovascular disease as assessed by medical history and did not take any medications. None of the participants had a history of smoking or regular physical exercise in addition to normal living. This study was reviewed and approved by the Institutional Review Board. Additionally, all procedures conform to the ethical guidelines of Helsinki Declaration. All potential risks and procedures of the study were explained to the subjects, and they gave their written informed consent to participate in the study.
Experimental protocol
The subjects abstained from alcohol, caffeine intake, and vigorous exercise for at least 12 h before the experiments. The experiment which consisted of 50-min intervention (leg heating or control) and pre- and post-intervention hemodynamics measurements was conducted in a temperature-controlled room (temperature ≈ 25 °C). Subjects remained supine position on a bed throughout the entire experimental protocol including at least 30 min of quiet rest before the start of pre-intervention measurement. Heat stimulation on both lower legs (under knees) was 20 min of exposure to far infra-red radiation at 43–45 °C (Leghot, Fujika Co., Ltd., Tokyo, Japan) followed by retaining warmth by a blanket for 30 min.16 In the resting control condition, subjects remained supine resting without the leg heating intervention. These trials were performed on separate days within a week using a crossover design (Fig. 1).

Experimental protocol.
Measurements
Heart rate, local (brachial and ankle) blood pressure (BP), and central (carotid-femoral) and leg (femoral-ankle) pulse wave velocities (PWV) were measured by a semi-automated vascular testing device (Form PWV/ABI: Model BP-203RPEII and TU-100; Colin Medical Technology, Komaki, Japan), as previously described.17 The central to leg arterial stiffness gradient was evaluated by subtracting central PWV from leg PWV. Central (aortic) hemodynamic variables were synthesized from applanation tonometrically-radial arterial pressure waveforms with the validated general transfer function-based pulse wave analysis software (SphygmoCor version 8.0, AtCor Medical, Sydney, Australia), as we previously reported.18 Aortic AP was defined as the difference between the first systolic peak (or shoulder) and second systolic peak pressures. Aortic AIx was calculated as the percent ratio of aortic AP to aortic pulse pressure, and standardized for a heart rate of 75 bpm. The timing of reflected wave return (TR) was calculated as the time from the beginning upstroke of the central pressure wave to the systolic upstroke of the reflected wave (inflection point). Synthesized aortic pressure waveform was decomposed into the forward (i.e., incident) and backward (i.e., reflected) waves using a triangular flow wave method validated by Westerhof et al.19 The amplitude and area under the curve were computed regarding the incident and reflected waves, respectively.
Statistical analyses
The effects of leg heating on hemodynamic variables were evaluated by repeated-measure ANOVA in a general linear model. In the case of a significant F value, Fischer’s LSD post hoc test was used to identify significant differences among mean values. All data were reported as means ± SEM. Statistical significance was defined a priori at P < 0.05.
Results
Hemodynamic responses to the intervention are presented in Table 1. There were no significant differences in all baseline variables between two trials. Heart rate and local (brachial and ankle) BP remained unchanged after the intervention in both trials. As shown in Fig. 2, leg PWV, but not central PWV, was significantly decreased following the leg heating (884 ± 19 vs. 851 ± 15 cm/s, P < 0.001). Subsequently, central-to-leg arterial stiffness gradient was markedly changed from positive to negative (+21 ± 33 vs. −33 ± 34 cm/s, P < 0.001; Fig. 3). In the control trial, no significant changes were observed throughout the experiment.
Variables | Before | After | |
---|---|---|---|
Heart rate, bpm | Control | 62 ± 2 | 59 ± 2 |
Leg heating | 63 ± 2 | 61 ± 2 | |
Brachial SBP, mmHg | Control | 125 ± 4 | 128 ± 4 |
Leg heating | 124 ± 4 | 124 ± 3 | |
Brachial DBP, mmHg | Control | 74 ± 2 | 76 ± 2 |
Leg heating | 73 ± 2 | 74 ± 2 | |
Brachial PP, mmHg | Control | 51 ± 2 | 52 ± 2 |
Leg heating | 50 ± 2 | 50 ± 2 | |
Ankle SBP, mmHg | Control | 144 ± 4 | 147 ± 4 |
Leg heating | 142 ± 4 | 143 ± 3 | |
Ankle DBP, mmHg | Control | 76 ± 2 | 77 ± 1 |
Leg heating | 75 ± 1 | 73 ± 2 | |
Ankle PP, mmHg | Control | 68 ± 3 | 70 ± 3 |
Leg heating | 67 ± 3 | 69 ± 3 |
Data are mean ± SEM. SBP, systolic blood pressure; DBP, diastolic blood pressure; PP, pulse pressure.
Heart rate and local blood pressure responses to the intervention.
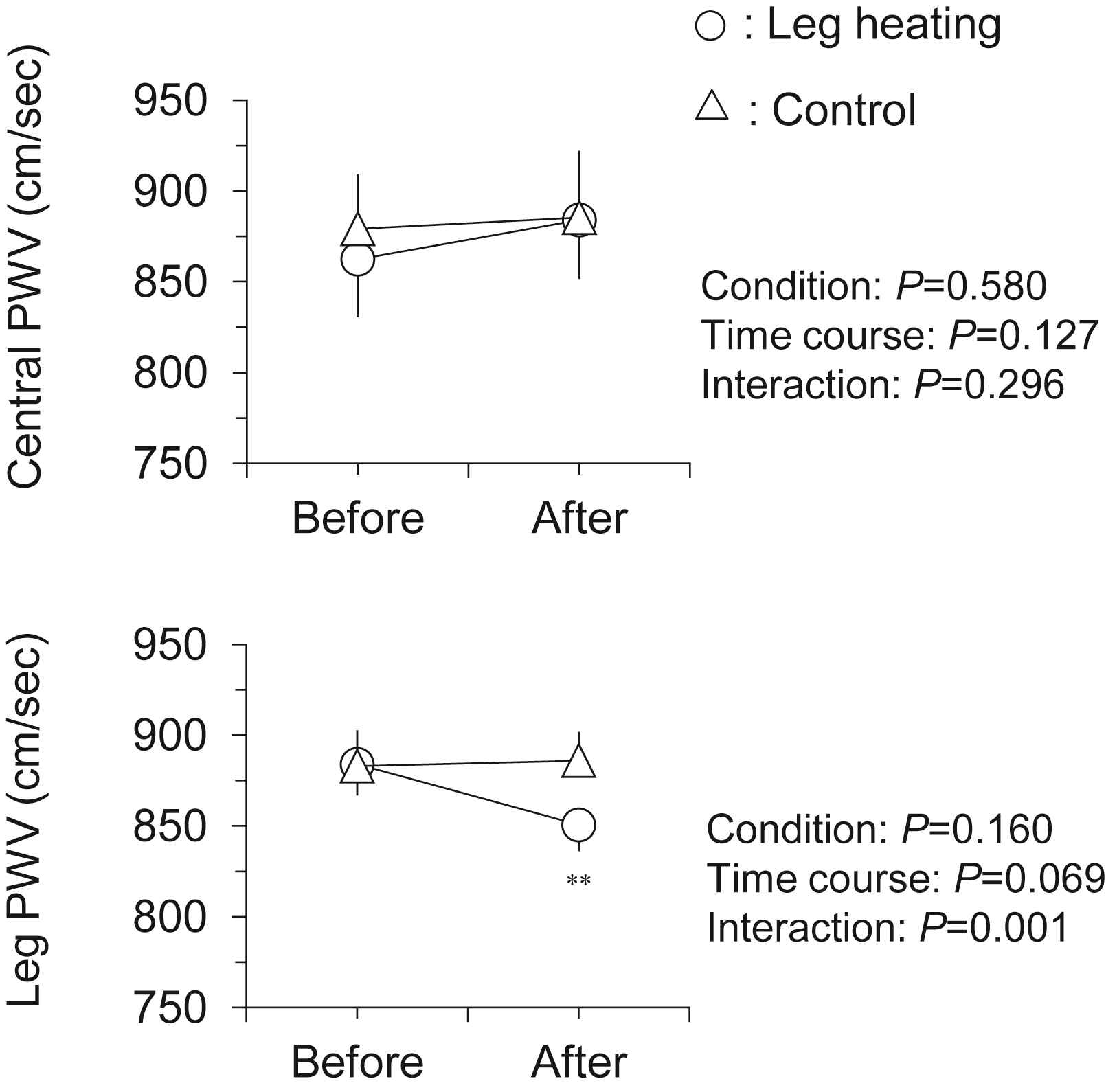
Changes in central and leg pulse wave velocity (PWV) with the intervention. Data are means and SEM. **P < 0.001 vs. Before.
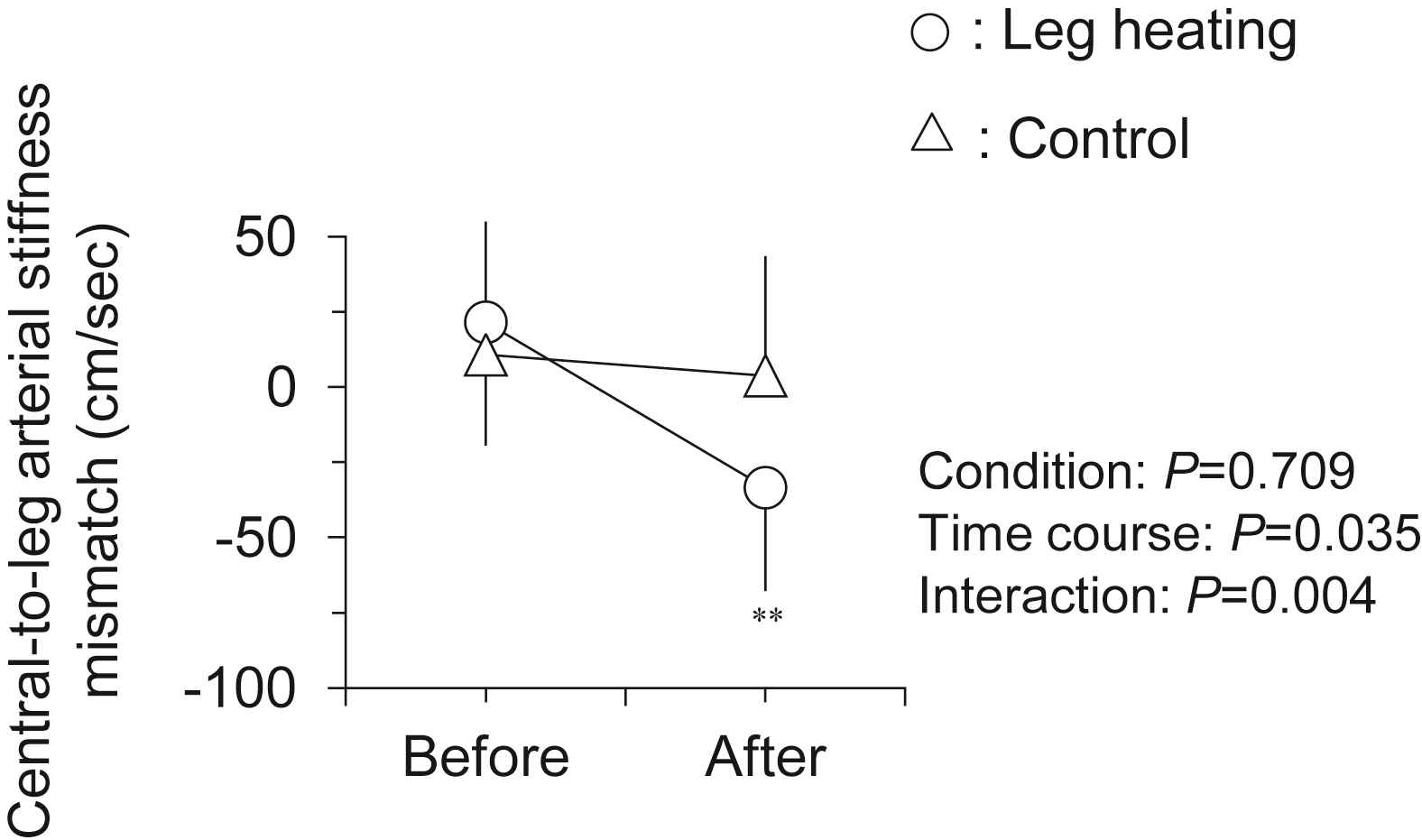
Changes in central-to-leg arterial stiffness mismatch (i.e., leg PWV – central PWV) with the intervention. Data are means and SEM. **P < 0.001 vs. Before.
Central hemodynamic measures before and after the intervention were summarized in Table 2. All measures were not changed significantly after the leg heating and resting control.
Variables | Before | After | |
---|---|---|---|
Aortic SBP, mmHg | Control | 119 ± 4 | 122 ± 3 |
Leg heating | 117 ± 4 | 118 ± 3 | |
Aortic DBP, mmHg | Control | 75 ± 2 | 77 ± 2 |
Leg heating | 74 ± 2 | 75 ± 2 | |
Aortic PP, mmHg | Control | 44 ± 2 | 45 ± 2 |
Leg heating | 43 ± 2 | 43 ± 2 | |
RWA, mmHg | Control | 18.7 ± 0.9 | 19.0 ± 0.9 |
Leg heating | 18.0 ± 0.9 | 17.7 ± 0.9 | |
TR, ms | Control | 136 ± 2 | 135 ± 2 |
Leg heating | 137 ± 4 | 138 ± 2 | |
Aortic AP, mmHg | Control | 12.5 ± 0.9 | 12.1 ± 0.9 |
Leg heating | 12.1 ± 1.1 | 11.1 ± 0.8 | |
Aortic AIx, % | Control | 30.7 ± 1.2 | 29.8 ± 1.2 |
Leg heating | 30.4 ± 2.0 | 28.4 ± 1.5 | |
Aortic AIx75, % | Control | 30.7 ± 1.2 | 29.8 ± 1.2 |
Leg heating | 30.4 ± 2.0 | 28.4 ± 1.5 |
Data are mean ± SEM. SBP, systolic blood pressure; DBP, diastolic blood pressure; PP, pulse pressure; RWA: reflected wave amplitude; TR, time of reflected wave return; AP, augmentation pressure; AIx, augmentation index; AIx75, augmentation index normalized by 75 bpm of heart rate.
Aortic hemodynamic responses to the intervention.
Discussion
Main findings of this study are as follows. A single bout of leg heating reduced the leg PWV but not central PWV. And thus, the central-to-leg arterial stiffness gradient was changed from positive to negative; nevertheless, central hemodynamic measures remained unchanged. These results suggest that the acute leg heating does not influence central pressure augmentation in postmenopausal women.
Contrary to the traditional wave theory, recent studies suggest that the reflected wave component of arterial hemodynamics does not contribute to augmented central pressure.11,12 Furthermore, it has been also reported that the reflected wave might become diminished until in the proximal aorta, and it no longer possible to identify contributions to central pressure augmentation.20 Thus, the determinants responsible for increasing central pressure augmentation and the precise locations of the wave reflection remain highly controversial, however, a widely held viewpoint is that, in the arterial tree, wave reflections occur at locations of impedance mismatch. Among various factors that are known to influence the intensity and timing of arterial wave reflection, the peripheral vascular tone of the lower body may have an important role of buffering or entrapment of forward travelling wave because of the fact that the lower body vasculature is characterized by a considerable degree of arterial branching and structural changes. Thus, we hypothesized that the lower leg heating changes the amplitude of reflected wave and central pressure augmentation via less central-to-leg arterial stiffness gradient as well as vasodilatation of leg microvasculature.
As intended, the lower leg heating decreased leg PWV, indicating the reduction of leg vascular tone. Moreover, the gradient of central-to-leg arterial stiffness changed from positive to negative because of no change in central PWV. Nevertheless, on contrary to our hypothesis, lower leg heating did not result in significant changes in central hemodynamics including the reflected wave and central pressure augmentation.
The fact that substantial change in the gradient of central-to-leg arterial stiffness did not result in the attenuation of reflected wave may imply that major wave reflection occurs more proximal sites including branches of renal arteries and aortic bifurcation rather than femoral bifurcation on contrary to traditional wave theory. Davies et al. suggested that reservoir function rather than wave reflection changes markedly with aging, and this is the major factor accounting the age-related changes in morphology of the aortic pressure waveform.6 Otherwise, wave reflections may mainly emanate in high-resistance arterioles.21,22 In this study, however, the size of dilated vascular beds (lower leg) might not be large enough to trap forward traveling wave effectively. Further investigation using the whole leg heating should be warranted.
Several methodological concerns should be mentioned. Firstly, since we had not measured the skin and core body temperatures, the degree of heat stimulation using the present study cannot compare with the other studies. Secondly, femoral-ankle PWV provides not the local but the averaged condition of whole leg vasculature. The interaction between changes in local hemodynamic measurements (e.g., femoral arterial impedance and lower leg vascular conductance) and systemic hemodynamics should be evaluated in the future study. Finally, as we did not conduct the measurements over a specific time-period, the time course changes of hemodynamics by the intervention are unclear.
In conclusion, the acute lower leg heating does not influence central pressure augmentation in postmenopausal women. Further studies gaining the mechanistic insight to account for the phenomenon are needed to specify a useful target for future prevention and therapy of CVD. Additionally, the present study only examined the acute effect of the leg heating. For clinical application, the chronic effects of the leg heating should be investigated in future.
Conflict of interest statement
The authors declare no conflict of interest.
Author contributions
K.K. and J.S. drafted the manuscript. K.K., J.S., and S.M. contributed to the interpretation and discussion of the results. K.K., J.S., and A.Z-M. contributed data acquisition and analysis. J.S. and S.M. provided the concept and design of the study and critically revised the manuscript.
Acknowledgement
We thank Dr. Nobuhiko Akazawa, Dr. Tomoko Matsubara, and Mrs. Yuriko Sawano for their technical assistance.
References
Cite this article
TY - JOUR AU - Keisei Kosaki AU - Jun Sugawara AU - Asako Zempo-Miyaki AU - Seiji Maeda PY - 2018 DA - 2018/02/12 TI - Impact of leg heating on central hemodynamics in postmenopausal women JO - Artery Research SP - 53 EP - 57 VL - 21 IS - C SN - 1876-4401 UR - https://doi.org/10.1016/j.artres.2018.01.001 DO - 10.1016/j.artres.2018.01.001 ID - Kosaki2018 ER -