Discussing the Need to Manage Uncertainty Relating to Users in Road Tunnel Fire Risk Assessment
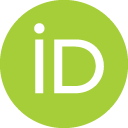
- DOI
- 10.2991/jracr.k.200507.002How to use a DOI?
- Keywords
- Fire; risk; safety; tunnel; users; uncertainty
- Abstract
Although tunnels provide roads with numerous advantages, they also include significant risks. Especially, fire accidents are the biggest threats. Nowadays, risk assessment has the predominant role for managing tunnel fire safety and users are in the centre of attention. However, existing methods exhibit lack in treating uncertainty stemming from users. This paper aims to discuss current ways of representing tunnel users’ uncertainty as well as to introduce the importance of distinguishing these representations in the wider context of risk assessment.
- Copyright
- © 2020 The Authors. Published by Atlantis Press SARL.
- Open Access
- This is an open access article distributed under the CC BY-NC 4.0 license (http://creativecommons.org/licenses/by-nc/4.0/).
1. INTRODUCTION
Although studies indicate the significant growth of maritime and the rise of intermodal transportation during the last decade, they also pinpoint that road transportation continues to hold the largest share in the transportation sector worldwide, having a considerable margin from the rest [1]. In that view, modern roads face the challenge to provide an adequate, intelligent as well as safe road network. Therefore, road infrastructure must be formed in order to cope with the arisen challenges. Although each part of the infrastructure should fulfill these challenges, special emphasis should be primarily given on its critical elements, such as tunnels [2].
Nowadays, tunnels have been an essential part for road networks to successfully meet these challenges. In general, the use of tunnels is strongly linked to the particular operational characteristics of the road network. To this respect, tunnels are divided into two major types. In summary, tunnels in urban areas aim to enable transportation flow in these areas by easing traffic congestion. In doing so, they also contribute to the enhancement of environmental quality of densely populated areas. On the contrary, the significance of tunnels belonging to rural areas and motorways is related to the reduction of travel time and cost commonly by creating shortcuts in mountainous areas [3].
Recent studies have shown that the number of tunnels is rising over the last two decades [4]. This progress has been supported by the enhancement of the mining technology, (e.g. improvement of tunnel boring machines), which has made tunnels become an attractive cost-effective engineering solution [5]. As a result, the share of both passenger and freight transport passing through tunnels is continously increasing.
As far as the safety issue is concerned, it has been named as the major challenge in the operation of road networks [6]. Therefore, each element of the road infrastructure should be characterised by a high safety level. Although tunnels do provide roads with numerous advantages, they also include significant risks for the operation of road networks. In particular, the type of accidents that can result in fire are the biggest threats [7]. The reasons are the impact of these accidents not only on the tunnel system itself but also on the rest road network. The devastating experience from fire accidents in the past has been didactic of the severity of such accidents [8]. The increase in the number and the length of tunnels implies that fire accidents will remain a substantial threat for each road network in the future. Thus, the need to adopt efficient safety strategies should be of high priority.
Given that an efficient fire safety strategy is related to the loss reduction rate succeeded in case of a fire accident, users are in the centre of attention when designing fire safety strategies for road tunnels. Nowadays, risk assessment has the predominant role for managing fire safety in road tunnels [9]. Risk assessment enables the safety analyst to estimate adequately potential losses amongst tunnel users in order to obtain an accurate description for the system’s level of safety. But, capturing and examining human behaviour in fires is a difficult task in every risk assessment process due to the significant uncertainty embeds. Road tunnels do not constitute an exception. However, existing risk assessment methods exhibit significant lack in dealing with tunnel users’ uncertainty in a rigorous way. Ntzeremes and Kirytopoulos [10] study has illustrate that the absence on treating users’ uncertainty can raise serious questions on the credibility of risk assessment studies. Based on this study, only a small change, about 30s in the parameter of the beginning of the evacuation process can change the acceptability of the safety level of the tunnel. Therefore, the purpose of this study is to discuss current ways of representing tunnel users’ uncertainty as well as to introduce the importance of distinguishing these representations into aleatory and epistemic uncertainty in the wider context of risk assessment for road tunnels.
2. KEY FEATURES OF FIRE SAFETY APPROACH
Tunnels prove to be safer road sections compared to the rest elements of the road network. There are plenty of reasons to justify this outcome. In brief, tunnels are not affected by dangerous weather conditions because of their enclosed environment, i.e. rainfall or snowfall. Additionally, they commonly lack junctions, pedestrians, stopped vehicles, and advertisement signages, which distract driver’s attention [11]. All these elements have been highlighted either as root causes of accidents or as aggravating factors. However, the most important factor that makes tunnels be safer is the driving behaviour of users inside tunnels. Relevant studies have indicated that users are more cautious when passing through tunnels [12].
Despite the lower accident rate in tunnels, the severity level of potential accidents is much higher. As far as fire accidents is concerned, their rates are even lower in tunnels than in open roads. However, the facts do not allow any complacency on this issue. Indicatively, in Norway, the country with more than a 1000 road tunnels and with the longest ones, the vehicle fires in tunnels average up to 20 per year between 2008 to 2011 [13]. China, which due to the economic growth is enhancing its road network, has more than 15,000 tunnels with a total length above 16,000 kilometers. Nevertheless, 161 serious fire accidents have been recorded in Chinese tunnels over the last 15 years [14].
The severity of fires in road tunnels depends on their intrinsic particularities, which are [15]:
- •
The absence of natural light that hinders drivers’ visibility when entering or exiting a tunnel,
- •
The difference in pressure between tunnel portals together with the piston effect developed by the vehicles movement. Both of them impact on the movement of the air, and in many cases they hinder the successful operation of ventilation,
- •
The difficulties for emergency services to get close to the fire promptly, and
- •
The unique characteristics of fires in enclosed environments.
Modelling fires in tunnels is a complicated issue because the behaviour of fire depends on both the attributes of burning objects and the environmental conditions, which affected by the enclosed nature of tunnels [16]. Geometrical, construction and operational characteristics of tunnels, which have differences to one another, impact too. For instance, tunnels can be unidirectional or bi-directional, which affect the forced movement of the air. Additionally, they have different length or width, different structure, and different traffic conditions (e.g. average volume, permission of dangerous goods, etc.).
The aforementioned factors impact on both fire development and evolution. In general, the conditions created from tunnel fires shape a highly unsafe environment for trapped users because they are affected by the high radiation released and the propagated smoke. These conditions can be even worse especially during the first minutes of fire evolution because the emergency ventilation system could not manage to impede the fire plume (i.e. hot gases and smoke) spread upstream of the fire location, creating thus the well-known backlayering phenomenon [17].
As a result of the significant losses caused in the trans-Alpine tunnel accidents occurred between 1999 and 2001 in France (Mont Blanc tunnel), in Austria (Tauern tunnel), and in Switzerland (St. Gotthard tunnel), tunnel managers and safety analysts raised serious concerns on the safety of these infrastructures [18].
Before these tragedies, safety management in road tunnels was carried out based on each country’s “prescriptive” approach. On a nutshell, each tunnel’s infrastructure and facilities should comply with the prescriptive requirements had been imposed. More details about prescriptive requirements exist in PIARC [19] study. However, the adverse effects of the aforementioned accidents uncovered with emphasis the deficiencies of the “prescriptive” approach. Indicatively, deficiencies arose regarding the surveillance of tunnels since the absence of a single control room led to significant reaction errors or the absence of escape routes associated with safety shelters in order to head trapped users to the external environment, which led to lot of users trap in these and burnt [20]. The aftermath of these disastrous events was that tunnel systems have become complex infrastructures making safety a complicated task. Therefore, fire safety management should fulfill the developed requirements [2].
Indeed, tunnels are the most significant structural element of the road network [3]. A quick look on their structure, their facilities, and their equipment features this point of view. Indicatively, complex surveillance systems are installed for monitoring each tunnel section. Their role is to provide sufficient as well as direct information of an incident/accident, namely its location and its evolution, in order to enable tunnel operators to activate the appropriate response protocols. Surveillance systems include either closed circuit television for continuously supervising traffic flows and identifying potential incidents/accidents or include sensors for detecting smoke and temperature from potential fire accidents. Other advanced technical installations existed in road tunnels are the ventilation and the fixed firefighting systems. Their existence is considered vital because they can control drastically the evolution of a fire event, enabling tunnel users trapped inside the tunnel to successfully evacuate themselves and mitigating damage to tunnel’s structure and facilities.
In view of that, the systematic approach of risk assessment has been used in conjunction with the prescriptive approach. Risk assessment is the proper tool to assess the safety level of the tunnel since it facilitates the examination of specific accidents as well as the observation of potential residual hazards, while assesses their inherent characteristics [21].
Fire risk assessment framework, incorporated in the field of road tunnel safety, consists of five stages forming a repetitive circle (Figure 1):
- (i)
Establish context. This stage consists of the system definition, and the specification of risk assessment’s goals and criteria.
- (ii)
Identify hazards. This stage aims to discover and record the hazards that can cause, directly or combined with others, to critical events, like fires.
- (iii)
Calculate the risks.
- (iv)
Evaluate the risks based on the risk criteria in order to judge their significance.
- (v)
Treat the unacceptable risks.

The risk assessment process.
In the end, the process is repeated in order to re-assess the renewed safety level of the tunnel system.
Understanding the operation of tunnel systems is a substantial step in order to conduct risk assessment. To simplify this process, the safety elements circle has been introduced (Figure 2). By grouping the various tunnel system parameters based on source characteristics, five discrete elements are developed. These elements are:
- (i)
The Users, i.e. walking speed, behavioural intentions, etc.
- (ii)
The Facilities, i.e. emergency exits, mechanical ventilation, etc.
- (iii)
The Infrastructure, i.e. tunnel length, slope, etc.
- (iv)
The Vehicles, i.e. Heavy Good Vehicles (HGV), bus, etc.
- (v)
The Traffic, i.e. volume, etc.

Safety elements circle, adapted from Ntzeremes and Kirytopoulos [4].
The use of risk assessment has introduced also the notion of the holistic approach, which every safety plan should follow. Therefore, each standard or additional safety measure, apart from the requirements of the specific discrete element, should also take into account all the potential interdependencies with the others.
3. UNDERSTANDING TUNNEL USERS’ UNCERTAINTY AND LINK TO FIRE RISK ASSESSMENT
3.1. Types, Definitions and Measures
Provided that road tunnels are complex systems (refer to Section 2), safety analysts commonly deal with problems throughout risk assessment process that require decisions under conditions of uncertainty. In general, two types of uncertainty are featured in risk assessment with which safety analysts come up against [22]. On the one hand is the so-called aleatory uncertainty. This type of uncertainty is variability that stems from diversity and stochasticity. On the other hand, there is the epistemic uncertainty. Epistemic uncertainty derives from either the partial ignorance or systematic measurement error or lack of knowledge. This type of uncertainty is the most common in risk analysis [23]. Human behaviour is treated to be non-deterministically in nature. In addition, a lack of complete knowledge is also presented when modelling human behaviour. Thus, human behaviour is characterised by both types of uncertainty. Tunnel users do not constitute an exception. Surely, the lack of knowledge and ignorance is related to the definition of a problem, the following methodologies, and the nature of the outcomes. However, due to the complexity of problems, (evaluating tunnel users’ self-evacuation is such a problem), safety analysts unavoidably will need to make decisions under conditions of limited knowledge and resources, thus necessitating increased dependence on the proper treatment of uncertainty [23,24].
Given that uncertainty is a decisive factor for the analysis and evaluation of risks, safety analyst should have to both recognise and quantify uncertainty sources throughout the risk assessment process. In doing so, the framework of probability is just following in order to represent it [23].
Probability theory is regarded as the better tool for coping with aleatory uncertainty. Bearing in mind that this kind of uncertainty is variability stemming from diversity or stochasticity, probabilities do provide an adequate mathematical concept for representing random or uncertain quantities. However, there are different views on what is the best approach for describing uncertain quantities associated with partial ignorance or systematic measurement error or lack of knowledge. Subjective probabilities are often used for representing this type of uncertainty. Although a subjective estimate of this probability is used, with some additional effort, it can be treated as a random variable bounded using either probability intervals or probability bound analysis or bounds based on evidence theory or percentile ranges [23]. The better the enhancement of knowledge and the awareness is, the better the ranges of the probabilities are updated.
Tunnel users’ losses are reasonably regarded as the representative parameter that provides the preparedness of the system in confronting fire accidents [25]. Therefore, each risk assessment method focuses on estimating the possible losses amongst users and subsequently is interested in mitigating them. Mitigating the losses in road tunnels means to minimise as low as reasonably practicable the potential losses amongst the users being involved in the accident. The term “reasonably practicable” means that “the risk must be insignificant in relation to the sacrifice (in terms of money, time or trouble) required to avert it” or else “risks must be averted unless there is a “gross disproportion” between the costs and the benefits of doing so” [26; p. 91]. Meanwhile, risk assessment should also be considered as the method for describing, the safety analyst’s together with the rest stakeholders of the system, uncertainties [22].
3.2. Evacuation Process on the View of Uncertainty
Each of the elements of the safety circle (Figure 2) includes parameters characterised by uncertainty of both types (see Section 3.1). Although a few studies have dealt with uncertainty of certain parameters (for more details refer to Ntzeremes and Kirytopoulos [4]), little has been done regarding the users.
Users consist the most vulnerable factor of the system. They are the first who confront with the fire consequences and in most of the cases without being experienced in such critical events. Moreover, they do not have appropriate equipment with them and they do not have the education of other groups on how to react in critical situations, like the members of the rescue teams [4]. Furthermore, fire in tunnels has much different behaviour than fire in the open road (i.e. developing of backlayering phenomenon, etc., see Section 2). As a result, users trapped in the tunnel have to evacuate themselves immediately after the spark of the fire without any delay in the beginning of the self-evacuation process.
During their self-evacuation users at the same time receive, recognise and interpret cues that affect their decisions before beginning their self-evacuation process. Subsequently, once movement gets started on, relevant cues such as the information received by the control room, the mental state or potential influence amongst users along with the exposure to heat, smoke and toxic gases may influence user’s responses and in turn his/her movement time. All these factors characterised by high uncertainty should be taking into account from the analyst [27].
Undoubtedly time is the basic engineering measure of trapped-users’ evacuation for rescuing themselves making rational decisions. Therefore, risk assessment is primarily focused on calculating the time it takes for trapped users to evacuate the tunnel walking away from the fire environment and heading towards a safe place. A basic representation of the fire emergency timeline that depicts the critical human responses or behaviours that impact and contribute to the evacuation process is illustrated in Figure 3.
The majority of the evacuation models are used in order to calculate the time needed for trapped users to evacuate the tunnel walking away from the fire environment and heading toward a safe place as the emergency exit doors or the tunnel portals (it depends from both the location of the fire and the user) as soon as possible. An important prerequisite in this direction is the establishment of two basic time parameters, the Available Safety Egress Time (ASET) and the Required Safety Egress Time (RSET). ASET is defined as the time which is actually available for trapped users from fire sparking and the time point at which conditions become inadequate for human life, because of the high rates of pollutant concentration and radiation. On the other hand, RSET refers to the time that trapped users actually need for a successful outcome of their self-evacuation. The aim of the safety analysts is to achieve the ASET to be greater than RSET [28]. To do so, they have to translate the stages of users’ evacuation process (see Figure 3) into time required to perform.

Evacuation process, adapted from Ntzeremes [27].
Along with the stages of the evacuation process, substantial information in order to comprehend better users’ behaviour in fires can be found in the relevant theoretical framework. These theories try to interpret the judgements of the actors in a critical situation. Fridolf et al. [20] study summarises the most commonly used theoretical frameworks. All of them, feature emphatically the inherent variability derived from users during their self-evacuation process. These are:
- •
The behaviour sequence model, which considers that actions of users consist of four distinct phases, namely: receive cues, interpret cues, prepare actions, and act.
- •
The role–rule model, which considers that each user acts following the rules associated with his/her professional status,
- •
The affiliation model, which considers that a user moves towards familiar to him/her places, and
- •
The social influence model, which considers that the behaviour of the crowd influences the evacuation process of an individual user.
However, fire risk assessment seems to neglect the presence of uncertainty. Most of the methods as well as evacuation models make various assumptions about the behaviour of trapped users during their evacuation. These approaches although simplify the complicated nature of the problem, they also hide the variability existed in human behaviour. Additionally, the deterministic approach of each country-specific regulatory requirements can cause similar consequences [21]. Thus, the uncertainty included in users can lead to a considerable uncertainty of the whole safety approach. Hence, it is important to design models taking into account potential information or clues from both real accidents and studies about human behaviour in fire evacuation and adapting them to the models, accordingly. If so, the uncertainties regarding the overall level of tunnel safety could be diminished.
3.3. Critical Analysis and Suggestions
The need for predicting how users would react just in case a fire spark in a tunnel has emerged intensely over the last two decades (refer to Section 3.1). Post-accident reports were the most valuable sources of data [29], which indicated that many tunnel users did not behave appropriately during their evacuation. For instance, some remained in their cars expecting emergency services to rescue them or some others considered shelters of the tunnel as fireproof safe places and stayed inside these facilities [8]. Consequently, a significant number of projects and full- or small-scale experiments has been interested in examining evacuation behavioural intensions of tunnel users.
Reviewing the relevant to the subject of users’ self-evacuation behaviour literature, one can found a significant number of valuable contributions on it during the last two decades, mainly after the trans-Alpine accidents. These studies can be utilised by safety analysts in order to deal with existing uncertainties. Ultimately, their contributions could be valuable in the subjective estimation of aleatory probabilities as well as in the minimisation of the epistemic uncertainty.
The inherent variability derived from users during their self-evacuation process is emphatically featured considering the various theoretical approaches having developed (refer to Section 3.2). Moreover, various experiments have been highlighted by different approach each time. For example, users’ behaviour in the UPgrading of existing TUNnels (UPTUN) project is interpreted through a mixed theoretical approach synthesised by both the behaviour sequence and the role–rule model. Detailed information can be found in Papaioannou and Georgiou [30] study. In addition, the behavioural sequence approach combined with the affiliation model are used in Seike et al. [31] study. In this particular case, one of the aims was to measure the movement speed of users in a Japanese road tunnel filled with smoke. In the studies of Kinateder et al. [32] social influence approach is the instrument in order to examine users’ evacuation in road tunnels experiment with virtual fires. Furthermore, in Nilsson et al. [33] experiment carried out in Sweden, the importance of social influence is examined for the success of the self-evacuation process. Ronchi et al. [34] study examined in a virtual reality small scale experiment the factors that impact on the choice of evacuation strategy of users by taking into account both the affiliation approach (distance to familiar safe places) and social influence.
As mentioned in Section 3.2, time is the basic engineering measure of trapped-users’ evacuation for rescuing themselves. However, fire risk assessment seems to neglect the presence of uncertainty when this measure is calculated. PIARC’s [19] reports provide some clues about evacuation behaviour and walking speeds. In addition to these reports, some methods provide specific walking speeds of the trapped-users deterministically, and in relation to the developed tunnel environment [35], although in a qualitative correlation in between. Fridolf et al. [36] study is the most recent report on this issue. Although provisions enable safety analysts in represent users’ evacuation, their deterministic approach can hide or disregard several aspects of the natural variability of users’ behaviours. Besides, the majority of the methods either do not give any information on this issue or provide oversimplified assumptions on the behaviour of users. Ntzeremes and Kirytopoulos [4] refer on the deficiencies of the European methods in addressing this issue. Consequently, the effectiveness of fire risk assessment can be drastically limited.
Last but not least, studies confirm that the level of education of users can determine significantly their actions in such cases. For instance, the study conducted in the Benelux tunnel illustrated that users who did not have any knowledge about the safety facilities existed in the tunnel were far away from reacting according to the expected norms. In particular, some users (i.e. motorists) did not recognise the criticality of the event heading on the fire location [37]. Similar conclusion was drawn in Gandit et al. [38] study, performed in a tunnel located in the French Rhone-Alpes Region, where evacuation behaviours of participants proved to be far away from the expected ones. The ACTEURS project examined how awareness of users combined with successful communication channels can impact on the evacuation process decreasing the wrong behaviours [39].
4. CONCLUDING REMARKS
Bearing in mind that tunnels are critical elements of the road network, safety is recognised a major challenge for their operation. Although the official accident rates showcase that tunnels are safer road sections compared to the rest network and despite their numerous advantages, yet they include significant risks for the road network. In particular, the accidents that can result in fire are the biggest threats. The increase in the number and the length of tunnels implies that fire accidents will remain a substantial threat for each road network in the future. Thus, the need to design efficient fire safety strategies should be of high priority.
The ultimate goal of an efficient safety strategy in the event of a fire accident in a tunnel is to minimise as low as reasonably practicable the potential losses amongst the users being involved in the accident. Therefore, by employing an adequate risk assessment method, risk analysts focus on estimating the possible losses amongst users and subsequently are interested in mitigating these losses. Given that tunnels are complex systems, safety analysts commonly deal with problems throughout risk assessment process that require decisions under conditions of uncertainty, both aleatory and epistemic. Analysing users’ behaviour in a fire accident and especially their self-evacuation process for estimating tunnel’s level of safety constitutes such case.
Capturing and examining human behaviour is a difficult task in every risk assessment process due to the significant uncertainty embeds. Road tunnel users do not constitute an exception. However, fire risk assessment in tunnels seems to neglect the presence of users’ uncertainty. Thus, the uncertainty is increased that subsequently leads to a considerable uncertainty of the whole safety approach. Most of the methods as well as evacuation models make various assumptions about the behaviour of trapped users during their evacuation. These approaches although simplify the complicated nature of the problem, they also hide the variability existed in users’ behaviour, concealing thus the level of safety of the tunnel. As a result, the decision of the analysts and tunnel managers regarding the need for receiving or not additional safety measures can be misled. Similar results can cause the deterministic approach commonly used by each country’s specific regulatory requirements.
Hence, it is important to use risk assessment methods and design evacuation models by taking into account potential information or clues from both real accidents and studies about human behaviour in fire evacuation.
In particular, time is the basic engineering measure for estimating users’ evacuation. Therefore, risk assessment is primarily focused on calculating the time it takes for trapped users to evacuate the tunnel walking away from the fire environment and heading toward a safe place. The literature indicates that during their self-evacuation users at the same time receive, recognise and interpret cues that affect their decisions before beginning their self-evacuation process. Subsequently, once movement gets started on, relevant cues such as the information received by the control room, the mental state or potential influence amongst users along with the exposure to heat, smoke and toxic gases may influence user’s responses and in turn his/her movement time. All these factors characterised by high uncertainty, which should be taking into account from the analyst.
Critical analysis of the literature illustrated the various sources of uncertainty embedded in human behaviour during evacuation process. In doing so, it aims to discuss current ways of representing users’ uncertainty as well as to introduce the importance of distinguishing these representations into aleatory and epistemic uncertainty in the wider context of fire risk assessment for road tunnels. Thus, safety analysts and others relevant to fire risk assessment on road tunnels can be motivated to modify the current framework.
CONFLICTS OF INTEREST
The authors declare they have no conflicts of interest.
ACKNOWLEDGMENTS
We would like to express our gratitude to the editors and the two anonymous reviewers for both their time and the valuable comments provided that helped the improvement of this paper. This research has been co-financed by the
REFERENCES
Cite this article
TY - JOUR AU - Panagiotis Ntzeremes AU - Konstantinos Kirytopoulos AU - Vrassidas Leopoulos PY - 2020 DA - 2020/05/13 TI - Discussing the Need to Manage Uncertainty Relating to Users in Road Tunnel Fire Risk Assessment JO - Journal of Risk Analysis and Crisis Response SP - 12 EP - 18 VL - 10 IS - 1 SN - 2210-8505 UR - https://doi.org/10.2991/jracr.k.200507.002 DO - 10.2991/jracr.k.200507.002 ID - Ntzeremes2020 ER -