Body Fluid pH Balance in Metabolic Health and Possible Benefits of Dietary Alkaline Foods
- DOI
- 10.2991/efood.k.190924.001How to use a DOI?
- Keywords
- pH; interstitial fluid; food; diabetes; insulin resistance
- Abstract
Living cells have several mechanisms to avoid acidic conditions created by excess protons. The protons are extruded to the extracellular space via the plasma membrane by various types of transporters such as the Na+/H+ exchanger and monocarboxylate transporters, which hereby contribute to the exclusion of protons in the cells of metabolic tissues including the liver, skeletal muscle, and adipose tissue. Disturbances in the functioning of the transporters cause abnormal intracellular fluid pH of the cells and dysfunction of metabolic homeostasis, leading to the development of metabolic diseases and a decrease in physical fitness level. In addition to the intracellular fluid pH regulation, growing evidence shows that the fluid pH in the interstitial space around metabolic tissues is easily reduced due to weaker pH buffering capacity than that in the cytosol and blood circulation. Therefore, pH reduction in the interstitial fluid may cause the onset of metabolic dysfunction. In contrast, several dietary foods have direct and indirect benefits in maintaining the interstitial fluid pH to the normal range by improving buffering capacities, suppressing proton production, and activating proton transporters, which strengthen the effect of appropriate diet on metabolic health.
- Copyright
- © 2019 International Association of Dietetic Nutrition and Safety. Publishing services by Atlantis Press International B.V.
- Open Access
- This is an open access article distributed under the CC BY-NC 4.0 license (http://creativecommons.org/licenses/by-nc/4.0/).
1. INTRODUCTION
Protons (H+) are produced from organic acids produced in living cells, and determine body fluid pH (the concentration of protons) [1–5]. Lactic acid (lactate−/H+) is one of the major organic acid-producing protons and causes lower fluid pH [1]. Glycolytic metabolism of glucose and glycogen under anaerobic conditions generates lactic acid (lactate−/H+) in metabolic tissues such as skeletal muscles and adipose tissues. The pKa of lactic acid is ∼3.8 at 37°C [6], therefore the generated lactic acid exists in ionized forms as lactate anion (lactate−) and protons at ∼pH 7.4 (physiological conditions). In contrast to the utility of lactate as an energy substrate in aerobic metabolism system, as referred to in the concept “lactate shuttle,” the dissociated protons are inducers of the acidic state [7,8]. Thus, the intracellular fluid pH is decreased under conditions of poor oxygen supply and excess glycolysis [9,10]. In addition, ketone bodies are other major sources of protons. β-Hydroxybutyric acid (β-hydroxybutyrate−/H+) is a major ketone body and a metabolite of fatty acid in the liver. β-Hydroxybutyric acid exists in its ionized forms, β-hydroxybutyrate anion (β-hydroxybutyrate−) and protons, reducing the intracellular fluid pH [1,11,12].
The intracellular fluid pH of living cells is mostly maintained at alkaline levels by various systems (Figure 1). As typical examples, the bicarbonate–carbonate (
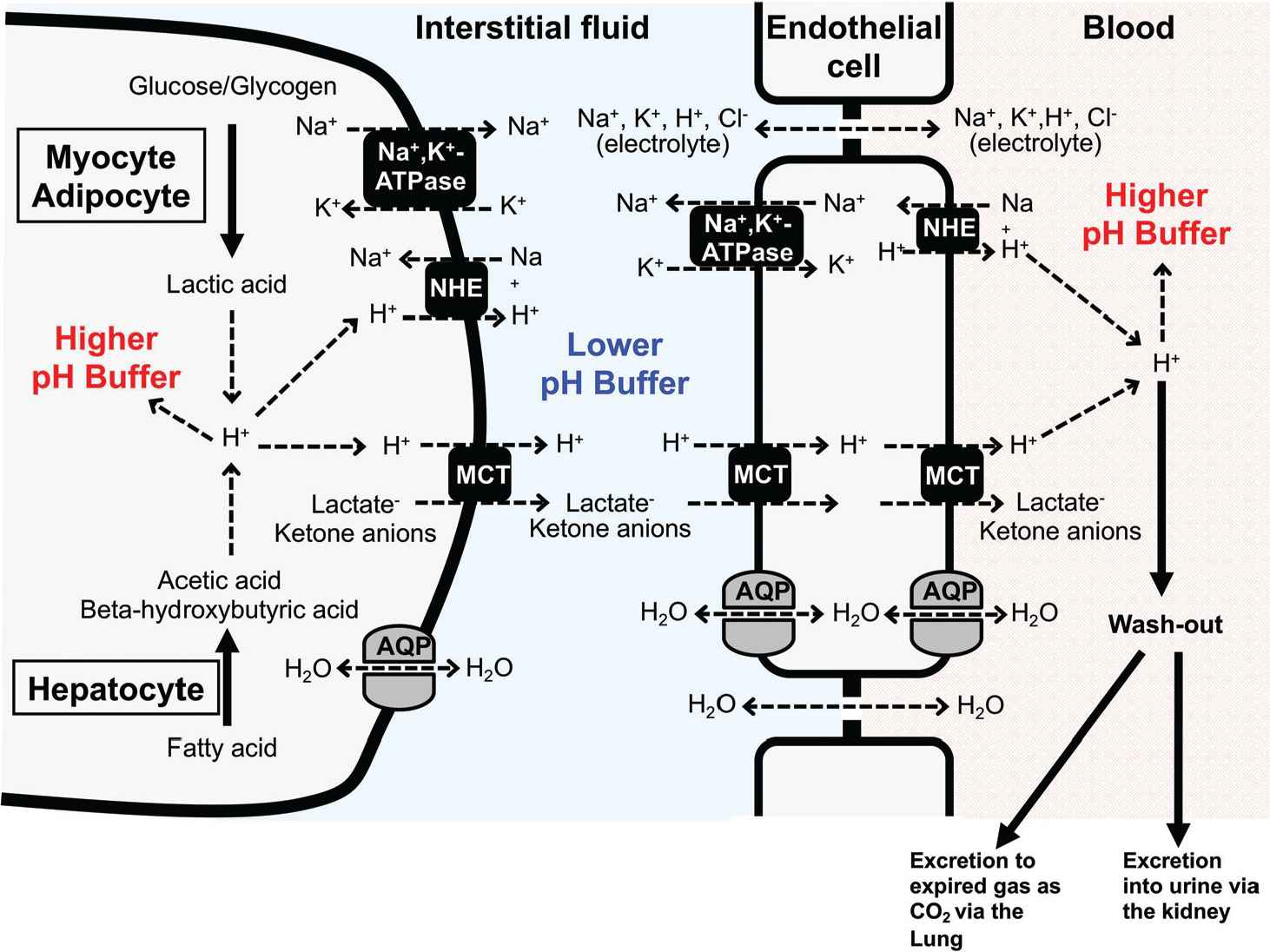
Cellular systems of pH homeostasis in metabolic organs. Elevation of glycolytic metabolism accelerates the production of lactic acid in both myocytes and adipocytes, whereas excess fatty acid metabolism in hepatocytes stimulates the production of ketone bodies, beta-hydroxybutyric and acetoacetic acids. The pH-buffering systems, proton efflux across the plasma membrane, and proton excretion strictly maintain intra- and extra-cellular pH. Monocarboxylate Transporter (MCT) and Na+/H+ Exchanger (NHE) participate in proton extrusion from the cytosol to the extracellular space. Further, proton was exported to circulation through transport systems on endothelial cells. However, in contrast to intracellular fluid and blood, the interstitial fluid could easily turn acidic due to poor pH-buffering factors such as proteins. AQP, aquaporin.
To maintain the intracellular pH in most living cells at alkaline levels as mentioned above, the acid produced in the intracellular space is extruded into the interstitial fluid (the extracellular fluid) [1,9]. Growing concepts suggest that the acidity of the interstitial fluid is one of the most serious pathogenesis causing various diseases including diabetes mellitus, tumor metastasis, and so on [1,9] (see the detail in Section 4).
Dietary intervention is a crucial lifestyle change required to maintain the acid–base balance in the body fluid [1–3]. Several food factors have potential roles in improvement of the buffering capacity and proton transport systems in the cells of metabolic tissues; thus, these factors may be useful in preventing metabolic dysfunction [1,2,4,5]. This review focuses on the significance of regulation of intracellular and extracellular fluid pH and the possible benefits of dietary foods associated with the prevention of disease development and improving physical fitness level.
2. BUFFERING AND EXCRETION OF PROTON IN pH REGULATION
Homeostasis of intra-body environments is the most important matter for keeping the body healthy. Regulation of body fluid pH is one of the most important key factors for maintenance of homeostasis by controlling various cellular and molecular functions such as the enzymatic reactions. Homeostasis of body fluid pH is mediated by various pH-buffering systems; for example, proton-binding proteins, such as albumin and hemoglobin (Hb), and proton-extruding transporters from the intracellular space to the extracellular one ultimately outside the body. However, when extremely large amounts of acids are produced, pH-buffering capacity is reduced, and/or proton-extruding transporting systems are impaired, the homeostasis of body fluid pH collapses, leading to health disorder.
Several buffering systems primarily contribute to the prevention of abnormal pH arising from excess proton production. A major buffering system is the bicarbonate–carbonate reaction in intracellular and extracellular fluids. The bicarbonate (
Proton transport across the plasma membrane is also important for maintaining the intracellular pH, particularly in the skeletal muscle, a major metabolic organ generating acids. In the contracting muscle, over 80% of the intracellular protons are transported from cytosol to extracellular space via the monocarboxylate transport system (MCTs), whereas the remaining protons are transported via the NHE,
Monocarboxylate cotransporters (a part of the solute carrier 16 family) cotransport monocarboxylate anions with proton across the plasma membrane. Different transport kinetics and location in 10 of the total 14 isoforms are characterized in Table 1. MCT1–MCT4 are mainly expressed in the cells of metabolic tissues such as skeletal muscle, adipose tissues, and the liver, and carry out the transport of lactate, pyruvate, and ketone bodies [14]. The direction of transport across the plasma membrane is dependent on the concentration gradient of protons and monocarboxylate [28–30]. When excess monocarboxylic acids are generated, MCTs play crucial roles in prevention against the acidic state. It has been known that the functional expression of MCT1 and MCT4 strongly affects lactate disposal in myocytes. MCT1 is highly expressed in the plasma membrane of slow twitch muscles [16,32,33]. In contrast, MCT4 is the predominant isoform found on the plasma membrane of fast twitch muscle, and transports lactate and proton from the cytosol to the extracellular space [31,33]. Another isoform MCT2 is mainly expressed on the plasma membrane of hepatocytes and exports ketone bodies to the extracellular fluid [34].
Isoform/Name | Major location | Major substrate |
---|---|---|
MCT1/SLC16A1 | Ubiquitous esp. erythrocyte, skeletal muscle (oxidative), heart, intestine | Lactate, pyruvate, butyrate, ketone bodies |
MCT2/SLC16A7 | Testis, spleen, brain, liver, kidney, pancreas | Pyruvate, lactate, ketone bodies |
MCT3/SLC16A8 | Retinal pigment epithelium, choroid plexus | Lactate |
MCT4/SLC16A3 | Skeletal muscle (glycolytic), leukocyte, astrocytes, chondrocyte, intestine, lung | Lactate, pyruvate, ketone bodies |
MCT5/SLC16A4 | Brain, muscle, liver, kidney, lung, placenta | Unknown |
MCT6/SLC16A5 | Kidney, muscle, brain, pancreas, prostate, lung | Drugs (bumetanide, nateglinide, probenecid) |
MCT7/SLC16A6 | Brain, pancreas, liver | Ketone bodies |
MCT8/SLC16A2 | Liver, heart, brain, thymus, intestine, ovary, prostate, placenta, skeletal muscle | Thyroid hormones |
MCT9/SLC16A9 | Endometrium, testis, ovary, breast, brain, retina | Carnitine |
MCT10/SLC16A10 | Kidney, intestine, skeletal muscle, heart | Aromatic amino acids |
MCT11/SLC16A11 | Skin, lung, pancreas, breast, ovary | Unknown |
MCT 12/SLC16A12 | Kidney, lung, retina | Creatine |
MCT 13/SLC16A13 | Bone marrow cells, breast | Unknown |
MCT14/SLC16A14 | Heart, brain, ovary, breast, pancreas | Unknown |
Characteristics of monocarboxylate transporter family
Na+/H+ exchanger also acts as a major proton transporter that maintains the intracellular fluid pH homeostasis. It extrudes the intracellular proton by taking in the extracellular Na+ using the Na+ chemical gradient between intra- and extra-cellular fluids [18,35]. NHE1–NHE5 of the total 10 isoforms are located on the plasma membrane of their specific tissues [35–37]. NHE1 is well known to act as a ubiquitous isoform and to contribute in the maintenance of pH homeostasis in metabolic organs.
3. pH DISTURBANCE IN PHYSIOLOGICAL AND PATHOLOGICAL CONDITIONS
The pH of arterial blood in mammals is strictly maintained within a range of 7.35–7.45 under physiological conditions. A decrease from the normal pH to <7.35 results in the condition of acidosis. During exercise, the elevated level of lactic acid production leads to the acidic state of the tissues and dysfunction of muscle contraction [38,39]. Although it depends on the intensity of exercise, strenuous contraction of the muscles can sometimes transiently reduce the pH of intramuscular fluid to ∼6.5 that is associated with >40 mM lactate accumulation in the intramuscular space [22,38,39]. In diabetic pathological condition, the body fluids of patients often show a chronic acidic state, called ketoacidosis, caused by excess production of ketone bodies [40,41]. The utilization of lipids instead of glucose for energy supply is accelerated in the liver, skeletal muscles, and adipose tissues with insulin resistance. The extreme large utilization of lipids (lipolysis) results in elevation of circulating fatty acids, causing the generation of ketone bodies via fatty acid oxidation in the liver. This process leads to high production of protons, ‘overloading protons’, which causes the metabolic ketoacidosis frequently observed in diabetic patients. The acidic conditions diminish activity of enzymes contributing to glucose metabolisms, such as phosphofructokinase, progressing pathophysiological conditions [40–42] and physical fatigue [43,44].
Loss of function of MCTs has been reported to disturb the body fluid pH homeostasis. The transport activity and substrate specificity of the MCT are influenced by various point mutations of the MCT gene. The mutation of arginine 306 to threonine in domain 8 of MCT1 spontaneously occurring has lower transport activity compared with the wild type MCT1 [45]. The mutated MCT1 with much lower transport activity delays recovery from high blood lactate after exercise [46,47]. A defect in lactate efflux from muscles due to the mutation of MCT1 would cause severe chest pain and muscle cramping after strenuous exercise. Furthermore, many differences in amino acids, not attributable to polymorphisms, are found in MCT1 obtained from muscle tissues in these subjects [46]. These findings suggest that mutations in MCT1 would be one of causes for physical fatigue and exercise performance. In contrast, the activity of lactate transport in muscle is also decreased by denervation and aging [48,49]. Dysfunction of MCT might be associated with metabolic disorder. The skeletal muscle of obese rats shows lower leveled expression of MCT1 and MCT4 than that in normal rats [50]. Notably, the study using heterogenous mct1-expressed mice (MCT1+/−) has shown a prevention in the development of diet-induced obesity and insulin resistance, suggesting that the MCT1 transporter can be a therapeutic target to prevent and treat obesity [51]. Further, this mutated mouse exhibited a higher acidic condition associated with lower carbonic anhydrase II contents [52], suggesting the important contribution of MCT1 in the acid–base balance of the body. In addition, the level of circulating lactate significantly shows a negative correlation to the degree of insulin sensitivity in humans [53,54], suggesting that insulin resistance would be caused by the lower lactic acid disposal caused by the reduction of MCT function.
4. INTERSTITIAL FLUID pH AND DISEASE DEVELOPMENT
Fluid pH in the interstitial space of metabolic tissues is easily reduced due to lower pH-buffering capacity than cytosol and blood circulation (Figures 1 and 2). The production of lactic acid is accelerated by elevating glycolytic metabolism in both myocytes and adipocytes [1] (Figure 1). In hepatocytes, the production of ketone bodies, beta-hydroxybutyric and acetoacetic acids, is accelerated by excess fatty acid metabolism [11,55]. Intra- and extra-cellular pH is strictly maintained by pH-buffering systems, proton efflux across the plasma membrane, and proton excretion [1] (Figure 1). Proton is transcellularly extruded from the cytosol to the extracellular space (the interstitial space) mainly by MCT and NHE (Figure 1). NHE, an electroneutral transporter, participates in H+ extrusion using the much higher chemical potential (concentration) of Na+ in the interstitial space than that in the intracellular space of myocyte, adipocyte, and hepatocyte even in the case of the higher H+ chemical potential (higher H+ concentration: lower pH) in the interstitial space than that in the intracellular space of myocyte, adipocyte, and hepatocyte. This means that NHE has potential to actively extrude H+ to the interstitial space from the intracellular space of myocyte, adipocyte, and hepatocyte (Figure 1) using the much higher chemical potential of Na+ in the interstitial space than that in the intracellular space. On the other hand, unlike NHE, MCT (an electroneutral transporter) has little high chemical potential for H+ extrusion from the intracellular space of myocyte, adipocyte, and hepatocyte to the interstitial one. The extrusion of H+, lactate and ketone anions to the interstitial space from the intracellular space of myocyte, adipocyte, and hepatocyte is driven by the summation of the chemical potentials of these anions (Figure 1). This means that in case of higher concentration of lactate and ketone anions in interstitial space than that in the intracellular space of myocyte, adipocyte, and hepatocyte, MCT could not actively extrude H+ to the interstitial space from the intracellular space of myocyte, adipocyte, and hepatocyte. In case the interstitial pH is lower than the intracellular pH of myocyte, adipocyte, and hepatocyte (the interstitial H+ concentration > the intracellular H+ concentration), lactate and ketone anions in the intracellular space of myocyte, adipocyte, and hepatocyte could not be actively extruded to the interstitial space. Therefore, to actively extrude lactate and ketone anions to the interstitial space from the intracellular space of myocyte, adipocyte, and hepatocyte, the interstitial pH should be kept higher than the intracellular pH of myocyte, adipocyte, and hepatocyte (the interstitial H+ concentration < the intracellular H+ concentration). The transport system of H+, lactate and ketone anions from the interstitial space to blood has an essential role in keeping the driving force for extrusion of H+, lactate and ketone anions via MCT from the intracellular space of myocyte, adipocyte, and hepatocyte (lowering the concentration of H+, lactate and ketone anions in the interstitial space; Figure 1). MCT expressed in the endothelial cell of blood vessels would participate in the transport of H+, lactate and ketone anions from the interstitial space to the intracellular space of the endothelial cells [56] (Figure 1). Further, MCT expressed in the endothelial cell also participates in the transport of H+, lactate and ketone anions to blood from the intracellular space of the endothelial cells [56] (Figure 1). NHE actively extrudes H+ from the intracellular space of the endothelial space to blood, keeping the low intracellular H+ concentration of the endothelial cell for the driving force of H+, lactate and ketone anion transport [56] (Figure 1). Thus, H+, lactate and ketone anions are transported from the intracellular space of myocyte, adipocyte, and hepatocyte to blood via the interstitial space. In addition to the transcellular transport pathway, the paracellular pathway is also available for the passive transport of small-sized electrolytes into blood from the interstitial space. The paracellular pathway is permeable to small-sized electrolytes, such as Na+, K+, H+, and Cl−. However, it is unclear how large the transport capacity of Na+, K+, H+, and Cl− is compared with that of the transcellular pathway. Therefore, in this review article, we just introduce that the paracellular pathway for the passive transport of small-seized electrolytes, such as Na+, K+, H+, and Cl−, is available across the interstitial space and blood.

pH-buffering systems in blood and interstitial fluids. Blood has strong pH buffers such as hemoglobin (Hb) and albumin, whereas interstitial fluids have weak pH-buffering molecules such as bicarbonate and phosphate molecules. This means that even under mild but not severe metabolic disorder conditions the arterial blood pH is maintained constant within the normal range (7.35–7.45), whereas the interstitial fluid pH becomes lower than the normal level. Modified from Figure 1 in Marunaka [1].
One of the most important points is that the interstitial fluid could easily turn to acidic condition due to poor buffering factors such as proteins in contrast to intracellular fluid and blood (Figures 1 and 2). Growing evidence suggests that the interstitial fluid acidosis could strongly contribute to the development of metabolic diseases. We have shown that before the development of diabetic symptoms, Otsuka Long-Evans Tokushima Fatty (OLETF) rats showed lower interstitial fluid pH in ascites and metabolic tissues than the normal pH (7.40) [57]. Notably, the interstitial fluid has little buffering capacity unlike the cytosol or blood that has a lot of proteins, strong buffering factors of proton [1,58,59] (Figure 2). The pH of blood (arterial blood pH) is strictly regulated within the range of 7.35–7.45 as blood has strong pH buffers such as albumin and Hb (Figure 2). On the other hand, interstitial fluids have only relatively weak pH buffers, bicarbonate and phosphate. Therefore, the pH of interstitial fluids has relatively variable pH values compared with the blood pH (Figure 2).
We have demonstrated, in an in vitro study, that low extracellular pH shows an inhibitory effect on the insulin signaling pathway in the L6 rat myotubes [60]. The binding affinity of insulin to its receptor, the insulin-induced phosphorylation level of insulin receptor, and the phosphorylation levels of Akt, a downstream target of the intracellular insulin signaling, are significantly diminished in lower extracellular pH along with the reduction in glucose uptake [60]. These in vitro observations indicate that insulin resistance would be caused by the low extracellular pH in the skeletal muscle cells. Therefore, the interstitial fluid pH in metabolic tissues readily changes (Figure 1) and may contribute to the onset of insulin resistance via diminution of insulin affinity to its receptor [1] (Figure 3). Other studies [61–63] have shown that the amount of organic acid production is correlated to insulin sensitivity in both type 2 diabetes patients and healthy subjects. A cross-sectional study of over 1000 subjects [61] has indicated that insulin sensitivity and urine pH are lower as body weight and waist circumference increase. The pH of 24-h urine in patients with metabolic syndrome was significantly lower than that in the normal subjects and the mean value of 24-h urine pH negatively correlates to the number of metabolic syndrome abnormalities [62,63]. Lower insulin sensitivity is observed in lower levels of serum bicarbonate and higher levels of anions resulting from metabolic acidosis [64]. Patients with obesity and type 2 diabetes show hyperlactacidemia [53], supporting that insulin sensitivity correlates to acidic condition. Even in healthy subjects, the urine acid level could be an independent risk factor for the development of type 2 diabetes [65].

Glucose metabolism impairment and interstitial pH reduction in skeletal muscle cells. Glucose and glycogen are used as major energy substrates. Excess glucose/glycogen utilization and aerobic metabolism impairment cause lactic acid production through the glycolysis pathway. Further, binding affinity of insulin to its receptor is diminished with pH reduction in interstitial fluid. This leads to suppression of insulin signaling activity and glucose uptake via Glucose Transporter 4 (GLUT4). Therefore, low interstitial fluid pH causes insulin resistance and hyperglycemia via diminution of insulin affinity to its receptor in skeletal muscle cells. IR, insulin receptor; IRS, insulin receptor substrate; PI3K, phosphoinositide-3-kinase; AS160, Akt substrate of 160 kDa; MCT, monocarboxylate transporter; NHE, Na+/H+ exchanger.
Hypertension, high blood glucose levels, visceral obesity, and dyslipidemia are frequently associated with insulin resistance, one of the major symptoms of metabolic disorders [66]. However, the mechanistic links between insulin resistance and hypertension is still unclear. Insulin resistance causes hyperinsulinemia due to insufficiency of insulin action in muscles with low pH of the interstitial fluid [1]. However, insulin effectively acts on its receptor in the kidney with normal pH of the interstitial fluid owing to rich blood flow [1], leading to an extensive reabsorption of Na+ via activation of epithelial Na+ channels activated by insulin [67]. The large amount of Na+ reabsorption causes volume-expanded hypertension [1]. Further, high intake of salt (NaCl) causes volume-expanded hypertension, decreasing the interstitial fluid pH due to large production of ATP spent for extrusion of Na+ from muscle cells (Figure 4). This drop of the interstitial fluid pH due to high intake of Na+ causes insulin resistance [1].

Possible molecular mechanism of the high salt intake-induced interstitial pH drop. High salt intake results in consumption of ATP required for the Na+, K+-ATPase for extrusion of the high-leveled intracellular Na+. To maintain the ATP level in the intracellular space, a lot of ATP should be produced associated with production of H+, reducing the interstitial pH. Modified from Figure 7 in Marunaka [1].
The high Na+ intake would cause an increase in the Na+ content in the interstitial space, elevating the volume of interstitial fluid via augmentation of the passive water movement into the interstitial space driven by an increase in the Na+-induced osmotic pressure of the interstitial fluid, and the expansion of the interstitial fluid volume disturbs the lymphatic flow resulting in a vicious circle associated with elevation of the interstitial fluid volume. The edema caused by other reasons, such as a low protein level in blood, hypertension, and so on, also shows a phenomenon similar to the high Na+-intake-caused edema. However, in cases of a low blood proteins and hypertension, the water movement between the inside of blood capillary and the interstitial is disturbed as described below. A low protein level in blood lowers the colloid pressure existing in the blood capillary, reducing the water movement into blood capillaries from interstitial fluid spaces across the walls of blood capillaries associated with disturbance of metabolite collection [68–71]. This means that if the interstitial fluid has strong pH buffers, such as proteins, the driving force of water movement into blood capillaries from interstitial fluid spaces reduces, resulting in edema. On the other hand, hypertension increases the water movement from blood capillaries into interstitial fluids across the walls of blood capillaries, resulting in edema [70]. Further, we should consider the paracellular diffusion caused by the leakiness of the blood capillary wall, leading to an increase in the protein content in the interstitial fluid associated with expansion of the interstitial fluid volume, the edema [70]. The pH regulation of interstitial fluid should be discussed carefully considering the cause of the edema. In cases of low blood proteins and hypertension, the composition of interstitial fluids would not be drastically changed as only the driving force of water movement changes with little change of permeabilities to solutes between blood and interstitial fluids including pH buffers such as bicarbonate and phosphate. Therefore, the pH regulation of interstitial fluids would not be drastically changed under the edema caused by low blood proteins and hypertension. On the other hand, in case of edema caused by the leakiness of the blood capillary wall, proteins such as albumin in blood would appear in the interstitial fluid, elevating the pH buffer capacity. Therefore, the pH regulation of interstitial fluids would be more strictly conducted.
Insulin resistance is the most important pathogenesis of type 2 diabetes and also develops cancer and cardiovascular diseases [72,73]. Thus, the fluid pH disturbance can cause abnormal metabolic regulation in a predisease state. Our observation [74] indicated that diabetic OLETF rats (26 weeks of age) show a lower interstitial pH than normal Wistar rats around the hippocampus, an important region for memory [75]. A high risk of developing dementia and Alzheimer’s disease has been reported in diabetic patients [76] who might have experience of defective memory functions. Insulin has an essential role in survival of the neuron within central nervous system [77]. The deficiency of insulin action causes unstable fluctuating blood glucose levels, leading to apoptosis and energy starvation [78–80]. Further, the fluctuation of blood glucose levels also forms neurotic plaques and neurofibrillary tangles, which produce the hallmark lesions of Alzheimer’s disease, and alters acetylcholine levels in the hippocampus [78–80]. The findings mentioned above lead us to an idea that: (1) the interstitial fluid pH is an essentially important key factor for metabolic homeostasis and (2) the maintenance of the interstitial fluid pH within the normal range or the recovery of the interstitial pH to the normal range is one of the novel therapeutic targets for metabolic brain disorders.
5. pH REGULATION BY APPROPRIATE DIETS AND FUNCTIONAL FOOD FACTORS
It is widely known that appropriate diet is an important habituation for the prevention of onset and progression of noncommunicable diseases. The maintenance of fluid pH homeostasis can be involved in the diet-induced metabolic health. Several intervention studies in humans have shown that fruit and vegetable-rich foods improve the acid–base balance and raise the urinary pH; these foods are collectively referred to as the alkaline diet [81]. Originally, food with alkaline characteristics has been recognized to contain abundant alkaline ash generated by food combustion. Furthermore, it has been suggested as acid excretion level into urine because urinary acid reflects acid–base balance of fluid in the whole body. To evaluate alkaline function of foods, acid excretion level into urine has been expressed as the potential renal acid loads that are calculated using excretion amounts of sulfate, chloride, phosphorus, sodium, potassium, calcium, magnesium, and organic acids, estimated from consumed foods and body size [82]. Subsequently, various fruits and vegetables were categorized as lower potential renal acid load (PRAL) foods and could induce the body fluid to alkaline state (Table 2). In contrast, animal food products showed higher PRAL levels. In addition, several strategies for functional examination have been developed on the basis of the concepts mentioned above (Figure 5). Various bioactive factors present in natural foods such fruits and vegetables can have potential alkaline functions that contribute to pH homeostasis through several mechanisms (Table 2).
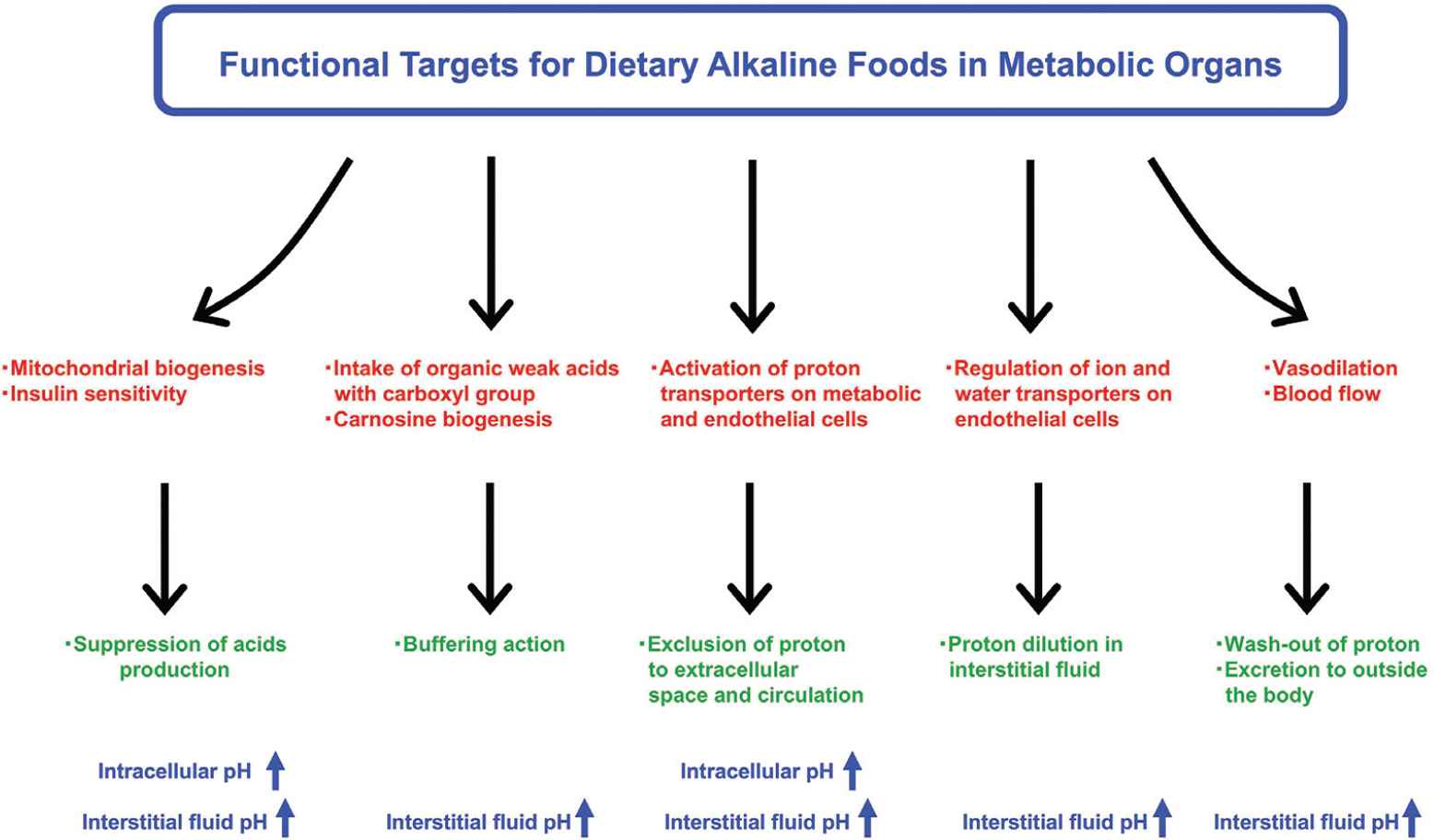
Research strategies for alkaline foods in metabolic organs. Bioactive factors contained in natural foods have potential benefits to prevent fluid acidification. Improvement of mitochondrial biogenesis and insulin sensitivity is one of the most important research strategies to suppress excess production of organic acids. Intake of organic weak acids with carboxyl group may also improve circulating and cellular pH-buffering capacities. In addition, acceleration of transporter-mediated proton excretion from cytosol to extracellular space across the plasma membrane on metabolic and endothelial cells can be another mechanism for preventing intracellular acidosis. Regulating water transport of the plasma membrane also contributes to the maintenance of pH homeostasis by diluting proton concentration in the fluid. Several food factors improve peripheral microcirculation through vasodilation, facilitating wash-out of proton for prevention of acidification.
Foods | Major nutrients/compounds | Function |
---|---|---|
Lower PRAL vegetables | ||
Spinach, celery, eggplant, tomato, green beans | Ascorbate, potassium, calcium, magnesium, flavone, β-carotene, lycopene | Reduction of acid load in the whole body |
Lower PRAL fruits and drinks | ||
Raisins, black currents, banana, apricots, orange, apple | Ascorbate, potassium, calcium, magnesium, anthocyanin, procyanidin, citrate, malate | Reduction of acid load in the whole body |
Red wine | Anthocyanin, resveratrol | |
Organic weak acid-rich foods | ||
Citrus, plum, vinegar | Citrate, acetate, malate | Buffering action |
Fermented foods | Propionate, butylate | |
Functional amino acid-rich foods | ||
Chicken, fish | β-alanine | Buffering action |
Soy, meat, fish, melon, watermelon, cucumber | Arginine, citrulline | Vasodilation |
Mitochondria activator foods | ||
Green tea, onion, apple, berry, red wine, salmon, shrimp | Catechin, quercetin, anthocyanin, resveratrol, astaxanthin | Reduction of acid production |
Potential renal acid loads of foods were evaluated based on the previous report [82]. Lower potential renal acid load (PRAL) foods were defined as lower than the value of −2.0. Mitochondrial activator food was defined as one that activates mitochondrial biogenesis and mitochondrial metabolic enzymes.
Major alkaline foods and functional compounds
A research strategy is to suppress excess production of lactic acids and ketone bodies by mitochondrial biogenesis for insulin sensitivity improvement in myocyte and hepatocyte. Several food factors including quercetin, catechin, anthocyanin, and astaxanthin enhance the Krebs cycle function by accelerating the entry of fatty acids from cytosol into mitochondria in the muscle cells. This is achieved through the elevation of activity and number of mitochondria in the muscle cells [83–87]. As the major energy substrates are carbohydrates and lipids, the lipid utilization compensates and decreases the utilization of carbohydrates, further decreasing the lactic acid production through the glycolysis pathway. On the other hand, enhancement of insulin sensitivity in metabolic organs maintains normal glucose metabolism. This leads to suppression of excess ketone body production from free fatty acids in hepatocyte, which also contributes to maintenance of pH homeostasis.
Circulating and tissue-buffering capacities are also improved by intake of organic weak acids such citrate, acetate, and malate [1] (see the detail shown in Figure 6). Indeed, dietary citrate has been shown to improve the circulating and intramuscular-buffering capacities [88,89]. The dietary organic acids are dissociated into anions and proton, and only the part of anions is absorbed from the intestinal lumen into circulation [1]. Thereafter, the organic anions function as pH-buffering factors in the peripheral tissues [1]. A dipeptide, carnosine (β-alanyl-
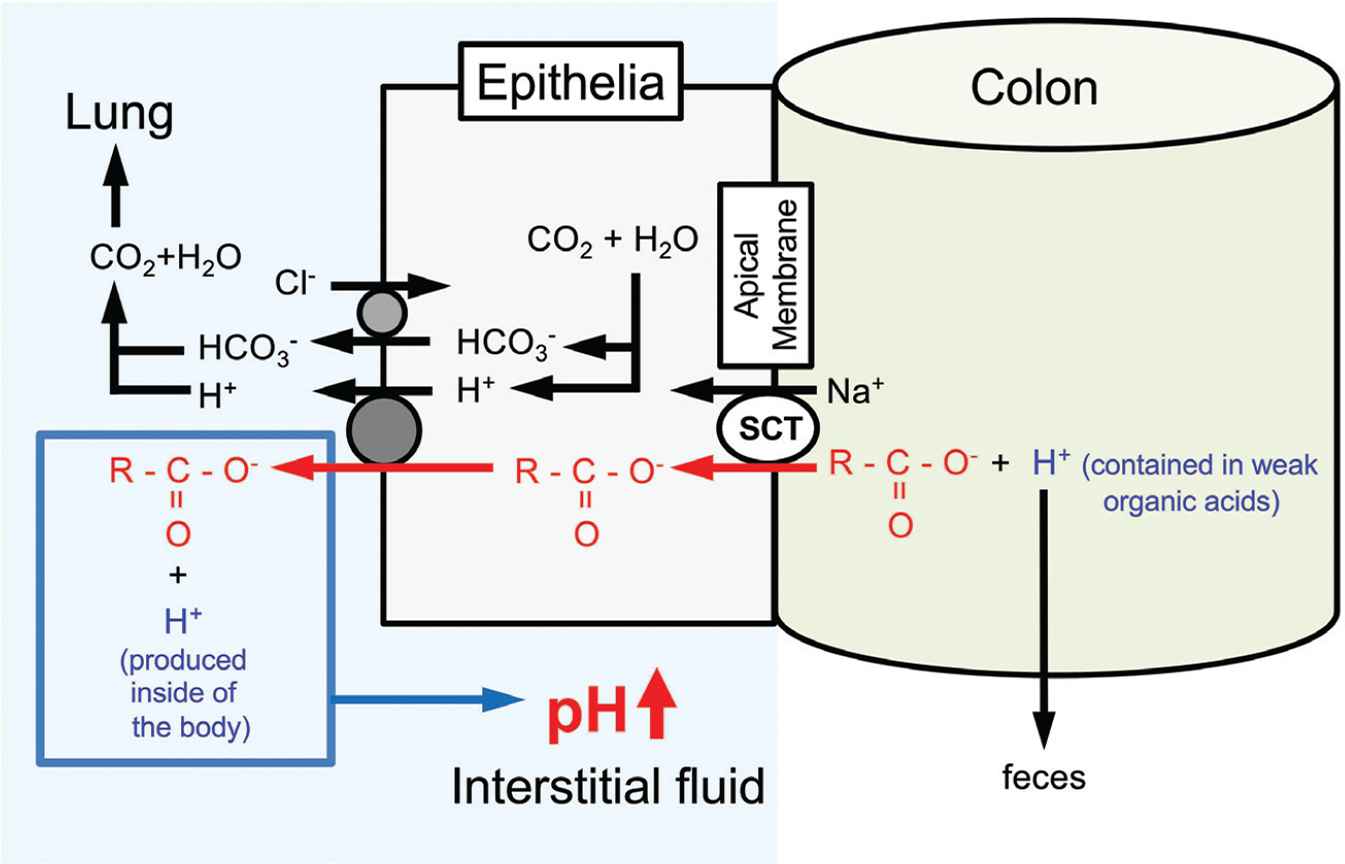
Action of oral intake of weak organic acids with carboxyl groups contained in foods on regulation of the interstitial fluid pH. By consuming weak organic ‘acid’ containing a carboxyl part (R-COO−) only the carboxyl part (R-COO−) is absorbed via sodium-coupled carboxylate transporters expressed in the apical membrane of the intestinal epithelium without H+ in the weak organic ‘acid’. Carboxyl groups incorporated into the intracellular space of the intestinal epithelium are transported from the intracellular space to the interstitial space (the extracellular space) of the intestinal epithelium via H+-coupled Monocarboxylate Transporters (MCT). H+ contained in weak organic acids is excreted into feces. Based on the phenomena, weak organic acids behave as ‘bases’ by combing with H+ produced in the body, elevating pH. This means that intake of weak organic acids elevates pH by increasing pH buffers in the interstitial fluid. Modified from Figure 5 in Marunaka [1].
Accelerating excretion of protons from cytosol to extracellular space via transporters located on the plasma membrane can be another mechanism for preventing intracellular acidosis. Several food factors improve peripheral microcirculation through vasodilation, resulting in facilitation of washout of proton. For example, arginine and citrulline amino acids promote nitric oxide production in endothelial cells in blood vessels, leading to the improved capillary circulation and acid removal [91]. Accelerating the excretion of protons from cytosol to the extracellular space or into circulation via transporters located on the plasma membrane can be another mechanism of the functional food factors for preventing intracellular acidosis. Many studies report that exercise intervention increases MCT1 and MCT4 levels in the skeletal muscle [92–95], whereas the effect of food factors on MCT or other proton transporters remains unclear. However, recent reports show that MCTs in skeletal muscle are upregulated by chlorella and black ginger extracts in animal and culture studies [96], suggesting the contribution of dietary foods in the maintenance of pH homeostasis via proton transport. In addition to the proton transport, dilution of proton concentration may contribute to maintenance of the interstitial fluid pH. Activation of water transport from circulation into interstitial fluid results in the proton dilution, leading to suppression of fluid acidification. However, as the premise, water transport depends on osmolytes such electrolytes and proteins contained in fluids because water is basically transported with osmotic pressure gradient between interstitial fluid and plasma. Therefore, it would be important to regulate both osmolytes and water transports. In the future, further diet intervention studies focusing on efficient proton and water transports should be developed.
Combined administration of food factors has more beneficial effects [97–99] with some evidence that supplementation of single nutrients could not clearly show their beneficial action on insulin resistance, cardiovascular risk, and physical performance [100,101]. The information suggests that intake of multiple nutrients would be more effective than that of a single bioactive factor. Propolis is a honeybee-collected natural product derived from plant resins. Various types of compounds are contained in propolis such as polyphenols, phenolic aldehydes, sesquiterpene quinines, coumarins, amino acids, steroids, and inorganic compounds [102]. Our previous report [57] indicates that propolis shows beneficial effects on metabolic disorders such as abnormal blood glucose levels in young (18 weeks of age) OLETF type 2 diabetic model rats, which show symptoms as type 2 diabetes mellitus such as obesity, hyperphagia, decreased glucose infusion rate in euglycemic clamp at 16–18 weeks of age, and hyperinsulinemia around 25 weeks of age responding to an intravenous glucose infusion [103,104]. Thus, our study [57] suggests that propolis is a compound showing beneficial, preventive action on symptoms of type 2 diabetes mellitus at early stages of the development of insulin resistance. Furthermore, this study [57] clearly reports that the pH of ascites and interstitial fluids around metabolic tissues is improved (elevated) by the intake of propolis compared with normal diet (without propolis intake), suggesting a possibility that dietary propolis suppresses the production of organic acids and/or elevates the pH-buffering capacity in those tissues. In addition, we have shown in a double-blind randomized placebo-controlled study in type 2 diabetic patients that the supplementation of propolis for 8 weeks has prevented the worsening of blood uric acid and improved the estimated glomerular filtration rate [104]. Therefore, propolis may be a useful compound to improve glucose metabolism associated with the prevention of acidic state. On the other hand, Japanese traditional herbal medicine is also known to improve various types of metabolic disorder symptoms including diabetes mellitus. Ninjin’yoeito (NYT), a Japanese traditional herbal medicine, consists of 12 species of crude drugs: rehmannia root, Japanese angelica root, atractylodes rhizome, poria sclerotium, ginseng, cinnamon bark, polygala root, peony root, citrus unshiu peel, astragalus root, glycyrrhiza, and schisandra fruit. NYT has also been shown to improve insulin resistance by elevating the interstitial fluid pH possibly via an increase in sodium-coupled monocarboxylate transporter 1 expressed in the colon, which transports only the part of carboxyl group without H+, leading to an increase in pH-buffering capacity [1,105,106] (Figure 6).
In the future, further studies should be conducted to gather more evidence on the effectiveness of alkaline foods against metabolic disorders like diabetes mellitus.
6. CONCLUSION
Regulating organic acid production and proton clearance is important for the prevention of acidic conditions in body fluids, and for the improvement of metabolic dysfunction and physical performance. Interstitial fluid pH, more than the intracellular and blood pH, can readily be reduced by acid stress, which may lead to the development of metabolic diseases. Appropriate diet and several bioactive factors have the potential to maintain pH, which might be associated with the activation of proton transporters, such as MCT and NHE, and to improve the pH-buffering capacity and blood flow. However, further studies are required to examine the contributions of various diets to pH homeostasis and metabolic health.
CONFLICTS OF INTEREST
The authors declare they have no conflicts of interest.
AUTHOR CONTRIBUTIONS
W.A. and Y.M. planned the whole designs of the article and wrote the manuscript. X.Z. and J.B.X. contributed to validation of the contents.
ACKNOWLEDGMENTS
This work was supported by Grants-in-Aid from
Footnotes
REFERENCES
Cite this article
TY - JOUR AU - Wataru Aoi AU - Xiaobo Zou AU - Jian Bo Xiao AU - Yoshinori Marunaka PY - 2019 DA - 2019/10/03 TI - Body Fluid pH Balance in Metabolic Health and Possible Benefits of Dietary Alkaline Foods JO - eFood SP - 12 EP - 23 VL - 1 IS - 1 SN - 2666-3066 UR - https://doi.org/10.2991/efood.k.190924.001 DO - 10.2991/efood.k.190924.001 ID - Aoi2019 ER -