CART Cell Toxicities: New Insight into Mechanisms and Management
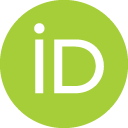
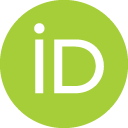
- DOI
- 10.2991/chi.k.201108.001How to use a DOI?
- Keywords
- CART cell therapy; Immunotherapy; Cytokine release syndrome (CRS); Neurotoxicity
- Abstract
T cells genetically engineered with chimeric antigen receptors (CART) have become a potent class of cancer immunotherapeutics. Numerous clinical trials of CART cells have revealed remarkable remission rates in patients with relapsed or refractory hematologic malignancies. Despite recent clinical success, CART cell therapy has also led to significant morbidity and occasional mortality from associated toxicities. Cytokine release syndrome (CRS) and Immune effector cell-associated neurotoxicity syndrome (ICANS) present barriers to the extensive use of CART cell therapy in the clinic. CRS can lead to fever, hypoxia, hypotension, coagulopathies, and multiorgan failure, and ICANS can result in cognitive dysfunction, seizures, and cerebral edema. The mechanisms of CRS and ICANS are becoming clearer, but many aspects remain unknown. Disease type and burden, peak serum CART cell levels, CART cell dose, CAR structure, elevated pro-inflammatory cytokines, and activated myeloid and endothelial cells all contribute to CART cell toxicity. Current guidelines for the management of toxicities associated with CART cell therapy vary between clinics, but are typically comprised of supportive care and treatment with corticosteroids or tocilizumab, depending on the severity of the symptoms. Acquiring a deeper understanding of CART cell toxicities and developing new management and prevention strategies are ongoing. In this review, we present findings in the mechanisms and management of CART cell toxicities.
- Copyright
- © 2020 International Academy for Clinical Hematology. Publishing services by Atlantis Press International B.V.
- Open Access
- This is an open access article distributed under the CC BY-NC 4.0 license (http://creativecommons.org/licenses/by-nc/4.0/).
1. INTRODUCTION
Immunotherapy has shown substantial promise as a cancer treatment. Significant clinical responses have been achieved through the genetic engineering of autologous T cells with chimeric antigen receptors (CARTs). In CART cell therapy, a patient’s T cells are extracted and modified ex vivo to confer greater potency and efficiency in attacking tumor cells. CARs are synthetic receptors made of an extracellular antigen-binding single chain variable fragment (scFv) domain joined to the intracellular signaling components of a T cell receptor [1]. CART cells are redirected to the tumor when the antibody-derived scFv binds to its cognate antigen on the cancer cell surface, which triggers T cell activation through the intracellular costimulatory and CD3ζ signaling domains of the CAR. The modular nature of the CAR enables targeting a broad range of tumor cell surface antigens by tailoring the antigen-binding domain on the CAR [2]. CD19 is an ideal antigen to target in B cell malignancies, as it is expressed on nearly all B cells and is not expressed on bone marrow stem cells, lowering the risk of off-target effects of anti-CD19 CART cell therapy [3].
The general method of manufacturing CART cells starts with the isolation and collection of a patient’s T cells via leukapheresis. The isolated T cells are activated with antibody-coated beads which serve as artificial antigen presenting cells. The activated T cells are genetically modified to express the CAR, most commonly through lentiviral transduction. The resulting CART cells are further expanded ex vivo. The patient typically undergoes a lymphodepleting regimen prior to CART cell reinfusion [4]. This is the framework most commonly used to develop CART cells, but there may be significant variations in manufacturing techniques.
2. CART CELLS IN THE CLINIC
T cells with chimeric antigen receptors cell therapy has led to exceptional success in treating certain hematological cancers, and the Food and Drug Administration (FDA) has approved three CART products, tisagenlecleucel, axicabtagene ciloleucel, and brexucabtagene autoleucel, for B-cell acute lymphoblastic leukemia (ALL), non-Hodgkin lymphoma, and mantle cell lymphoma, respectively. Real-world data are increasingly demonstrating the feasibility of CART cell therapy in patients otherwise ineligible for clinical trials and are signifying the need for novel strategies to mitigate CART-related toxicities [5]. Treatment of relapsed or refractory ALL has made progress over the years, with immunotherapies such as inotuzumab and blinatumomab showing promising results [6]. CART cell therapy has also displayed remarkable results in these patients, with complete remission rates as high as 90% [7]. CART cell therapy has also been successful in treating chronic lymphocytic leukemia (CLL) [8–10]. For the treatment of multiple myeloma (MM), CART cells directed toward B cell maturation antigen (BCMA) exhibited anticancer efficacy in early phase clinical trials [11,12]. Recently, it was stated that CART cells directed towards the activated integrin β7 can target and eliminate MM cells together with CD19+ B cells, and clinical trials have been initiated [13].
3. TOXICITIES OF CART CELL THERAPY
Despite the successes of CART cell therapy, treatment is limited by the associated toxicities of cytokine release syndrome (CRS) and immune effector cell-associated neurotoxicity syndrome (ICANS), both of which can be lethal [14,15]. CRS is an inflammatory condition linked to a rapid increase of activated T cells which results in significant serum elevation of cytokines including soluble IL-2Rα, IL-6, IL-10, and interferon gamma (IFN-γ) [16]. Manifestations of CRS can range from mild cold-like symptoms to severe multiorgan dysfunction. CRS typically develops within days to weeks after CART cell administration during peak T cell expansion [17]. Patients who experience severe CRS after CART cell therapy frequently show signs and symptoms of macrophage activation syndrome (MAS), with elevated ferritin levels and organomegaly [18].
Cytotoxic T cells, natural killer cells, and Th1 cells produce IFN-γ, an inflammatory cytokine involved in the differentiation of Th1 cells and activation of macrophages [19]. IFN-γ produced by CART cells may lead to secondary MAS seen in patients with severe CRS [7]. Although many IFN-γ inhibitors are clinically available, this potential treatment strategy for CRS may dampen CART cell efficacy, as IFN-γ is important to cytotoxic T cell activity. Better understanding of the underlying mechanisms of CRS will aid in the discovery of suitable cytokine targets to decrease toxicity while preserving CART cell efficacy.
Cytokine release syndrome is the main toxicity observed in ALL patients treated with CART cells [20]. There may be an association between CRS and CART cell efficacy: patients who experience CRS are more likely to respond to the therapy. However, there does not seem to be a strong association between the severity of CRS and response to treatment [7]. In a study by Hay et al. [21], CRS was frequently seen after treatment with CART cells, occurring in 70% of patients; however, most cases were mild to moderate and resolved without requiring tocilizumab or dexamethasone.
Immune effector cell-associated neurotoxicity syndrome is the second major toxicity that occurs in a significant number of patients who receive CD19-targeted CART cell therapy [22]. CART-associated ICANS most commonly manifests in aphasia, dysgraphia, lethargy, obtundation, and seizures [23,24]. CT and MRI have not been successful in clarifying the cause of ICANS, although CART cells can be visualized in the cerebrospinal fluid of many patients, irrespective of encephalopathy [7]. Severe ICANS develops most often in patients who experience CRS and almost always after an initial fever. Elevated levels of C-reactive protein and fever present earlier in patients with severe ICANS compared to those with mild ICANS [25,26]. Onset of ICANS can vary considerably and has been observed between 2 days to 4 weeks after CART cell infusion, necessitating close observation during the course of CART cell therapy [24].
An anticipated on-target toxicity of effective CART cell therapy is chronic B-cell aplasia and subsequent hypogammaglobulinemia. As CD19 is expressed on nearly all B cells, both healthy and malignant mature and developing B cells are eradicated by CD19-targeting CART cell therapies. Long-term CART cell persistence generally predicts better antitumor response, but also results in continued B-cell aplasia. Although immunoglobulin replacement alleviates many complications, monitoring is required to evaluate delayed toxicity of B-cell aplasia [27].
4. TOXICITIES IN DIFFERENT DISEASES
The incidence and severity of CART-associated toxicities vary by disease type and are summarized in Table 1. ALL patients tend to have higher rates of CRS (77–93%) [28–30] than lymphoma patients (37–93%) [31–34] after CART cell administration, and MM patients have the highest rates of CRS occurrence (76–94%) [35–38]. Regarding severe CRS (≥grade 3), the highest rates are seen in patients with ALL [28–30]. In the latter, the greater incidence of severe CRS may be due to disease burden and the aggressiveness of the cancer. Approximately half of the patients in early CART cell clinical trials needed interventions such as intravenous (IV) fluids, vasopressors, positive pressure oxygen, and hemodynamic monitoring due to CRS [16,31]. In one trial, approximately 30% of patients needed to be admitted to the intensive care unit due to hemodynamic and respiratory complications from CRS [16]. A greater understanding of CRS mechanisms and advancement in the treatment of CRS are needed to decrease these rates.
References | Disease type | CAR construct | Population | CRS | NT | CRS management |
---|---|---|---|---|---|---|
Maude et al. [28] | B-ALL | Anti-CD19 CART | N = 75 | 77% CRS | 40% NT | 37% Tocilizumab, NR% corticosteroids |
4-1BB | Peds & AYA | 46% severe | 13% Severe | |||
Gardner et al. [30] | B-ALL | Anti-CD19 CART | N = 45 | 93% CRS | 49% NT | 37% Tocilizumab, 23% corticosteroids |
4-1BB | Peds & AYA | 23% severe | 21% Severe | |||
Park et al. [29] | B-ALL | Anti-CD19 CART | N = 53 | 85% CRS | 62% NT | 11% Tocilizumab, 21% corticosteroids |
CD28 | Adults | 26% severe | 42% severe | |||
Schuster et al. [32] | B cell lymphoma | Anti-CD19 CART | N = 93 | 58% CRS | NR-NT | 15% Tocilizumab, 11% corticosteroids |
4-1BB | Adults | 23% severe | 12% severe | |||
Neelapu et al. [31] | B cell lymphoma | Anti-CD19 CART | N = 108 | 93% CRS | 67% NT | 45% Tocilizumab, 29% corticosteroids |
CD28 | Adults | 23% severe | 30% severe | |||
Abramson et al. [33] | B cell lymphoma | Anti-CD19 CART | N = 102 | 37% CRS | 23% NT | 17% Tocilizumab, 21% corticosteroids |
4-1BB | Adults | 1% severe | 13% severe | |||
Zhao et al. [35] | MM | Anti-BCMA CART | N = 57 | 90% CRS | 2% NT | 46% Tocilizumab, 11% vasopressor, and 35% supplemental oxygen |
4-1BB | Adults | 7% severe | NR-severe | |||
Brudno et al. [36] | MM | Anti-BCMA CART | N = 16 | 94% CRS | NR-NT | 31% Tocilizumab, 25% corticosteroids, 38% vasopressor |
CD28 | Adults | 38% severe | 19% severe | |||
Cohen et al. [37] | MM | Anti-BCMA CART | N = 25 | 88% CRS | 32% NT | 28% Tocilizumab, 21% corticosteroids |
4-1BB | Adults | 32% severe | 12% severe | |||
Raje et al. [38] | MM | Anti-BCMA CART | N = 33 | 76% CRS | 42% NT | 21% Tocilizumab, 12% corticosteroids |
4-1BB | Adults | 0% severe | 3% severe |
Different severity grading scales were used.
AYA, adolescent and young adults; Peds, pediatrics.
CRS and neurotoxicity rates
The appearance and incidence of ICANS differ between studies. ICANS rates for patients with ALL (40–62%) [28–30] or lymphoma (23–67%) [31,32,34] tend to be higher than those of MM patients (2–32%) [35–37]. Following similar trends, severe ICANS (≥grade 3) occurs in 13–42% of ALL patients, 12–30% of lymphoma patients, and 12–19% in MM patients. Contributing factors to ICANS include the type of malignancy, tumor burden, treatment history, and patient age [25,28,29], in addition to the CAR construct and dose administered [39].
According to the ZUMA-1 study, grade 3 or higher cytopenias that were not resolved within 3 months following anti-CD19 CART cell therapy were seen in 17% of patients [40]. Due to the possibility of persistent cytopenias, it is recommended to regularly monitor the patient blood counts after CART cell therapy [41].
5. MECHANISMS OF CART CELL TOXICITIES
Cytokine release syndrome and ICANS develop when large numbers of CART cells proliferate in the patient [29]. Greater peak numbers of CART cells can develop in patients with greater bone marrow disease burden, with higher doses of CART cells, or by preconditioning with fludarabine to allow improved CART cell engraftment [21]. Preconditioning chemotherapy with IL-7 and monocyte chemoattractant protein-1 (MCP-1) is associated with more robust lymphodepletion and better anti-tumor efficacy and has been shown to increase CART cell activity [42,43], in part due to the ablation of regulatory T cells and increase in cytokines such as IL-15. Moreover, lymphodepleting chemotherapy has also demonstrated improved CART cell persistence [44,45] Careful adjustment of the CART cell dose infused in patients with greater disease burden or with extensive preconditioning may reduce toxicities associated with CART cell therapy [46].
The mechanisms of ICANS are less understood than those of CRS, but insights have been made through retrospective analyses [47]. Higher grades of ICANS develop almost entirely in patients who have also experienced CART-related CRS [25,39], and ICANS may develop concurrently or after CRS onset [25,39]. Occurrence of severe ICANS has been linked to severe CRS, suggesting overlapping contributing factors of these two CART-associated toxicities [28,30,47].
The precise role of CART cells in the development of ICANS is uncertain, and CART cell presence in the cerebrospinal fluid (CSF) does not appear to correlate with ICANS [16]. However, patients experiencing ICANS have significantly elevated cytokine levels in the CSF, including MCP-1, inducible protein-10, IL-6, and IL-8 [25]. These observations suggest a role played by stimulated myeloid cells, particularly in the central nervous system, and a subsequent increase in blood–brain barrier permeability [26]. Higher levels of angiopoeitin-2, a cytokine involved in angiogenesis, were also observed in the circulation of patients with severe CRS and ICANS, suggesting that triggering endothelial cells may lie at the root of both of these conditions [21,39]. Additionally, elevated concentrations of glutamate, an excitatory N-methyl-D-aspartate (NMDA) receptor agonist, were discovered in patients with severe CART-associated ICANS, offering an explanation for the occurrence of seizures after CART cell administration [25]. Preclinical models have focused on the analysis of serum cytokine levels to predict the onset of extreme ICANS and may be useful in directing clinical trials. However, attaining cytokine levels in real time is challenging and limits the use of these models [25,39,44].
The type of costimulatory domain appears to have an impact on onset and severity of CART-associated toxicities. It has been suggested that there is a greater risk of ICANS with CAR constructs containing CD28, as seen when five instances of lethal cerebral edema occurred after CART cell therapy utilizing a CD28 costimulatory domain, resulting in the cessation of an ALL clinical trial [48]. However, similar severe adverse events have also developed with 4-1BB-costimulated CART cells [39]. Some studies reported greater rates of severe ICANS after CD28-CART cell administration [29,31], while other studies have reported lesser rates [49]. CRS develops earlier in patients with CD28-CART cells compared to patients receiving 4-1BB-CART cells, but definitive correlations between a specific costimulatory domain and the risk of CRS or ICANS do not currently exist.
6. GRADING OF TOXICITIES
The severity of CRS or ICANS is graded according to various guidelines, and identifying the discrepancies and synchronizing the grading schemes are crucial to facilitate better comparisons between clinical trials. The American Society for Transplantation and Cellular Therapy (ASTCT) issued a simplified CRS grading scheme in which fever ≥38°C is a criterion for diagnosis, and hypoxia and hypotension are the main determining factors of the consensus grading system [23]. In this current system, any need for vasopressor support is considered grade 3+ CRS, whereas CRS was previously classified as either grade 2 or 3 depending upon the dose of vasopressor. The scoring requirements for oxygen have also been simplified. Organ toxicities are no longer included in the CRS grading. These recent alterations have made the CRS grading system less complex and easier to compare between different studies.
A grading scheme for ICANS developed by the CAR T-Cell Therapy–Associated TOXicity (CARTOX) consensus group consists of a 10-point grading (CARTOX-10) combining important components of the Mini Mental State Assessment to assess the grade of encephalopathy by variations in concentration, speech, handwriting and orientation [15]. This grading system is more comparable between studies and has simplified the evaluation of encephalopathy. However, other characteristics of the CARTOX scoring system, such as papilledema measurements, were too unwieldly and imprecise to be widely implemented. The new ASTCT guidelines for assessing imICANS use previous grading schemes and offer a straightforward and more precise method to classify the severity of ICANS [23] by using immune effector cell encephalopathy (ICE) scores. ICE scoring is a newly modified encephalopathy screening method similar to the CARTOX-10 system, but it includes a component for evaluating receptive aphasia frequently observed in these patients.
7. MANAGEMENT STRATEGIES FOR CART-ASSOCIATED TOXICITIES
There have been significant improvements in the management of CRS and ICANS associated with CART cell therapy. Currently, the most commonly used strategies to manage CART-related toxicities are supportive care, tocilizumab and steroids (Table 2). With better understanding of the pathophysiology of CRS and ICANS through ongoing studies, future management strategies can be optimized.
CRS management strategy | Mechanism | Advantages | Disadvantages |
---|---|---|---|
Supportive care | Treats symptoms of hypotension and hypoxia with IV fluids, vasopressors, and positive pressure oxygen | Effective in maintaining organ function and preventing further damage | Does not address root cause |
Tocilizumab | Blocks IL-6-mediated effects; IL-6 is a key CRS-associated cytokine | Rapidly reverses CRS, targets specific cytokines involved in CRS cascade | Does not cross blood–brain barrier—ineffective for treating neurotoxicity |
Steroids | Nonspecific immunosuppression reduces inflammation | Effective in many tocilizumab-refractory CRS cases | Non-targeted immunosuppression may limit CART efficacy and persistence |
Commonly used management strategies for CART cell toxicities
7.1. Cytokine-directed therapy: IL-6, IL-1, GM-CSF
IL-6 is associated with severe CRS after CART cell therapy, and tocilizumab, a monoclonal antibody against IL-6R, was approved by the FDA for the management of CRS [50]. Tocilizumab had originally been used to treat rheumatoid and juvenile arthritis but has also shown effectiveness in reducing CRS-associated symptoms after the infusion of CART cells [18]. The dosage suggested by the FDA is 8 mg/kg for adult patients and 12 mg/kg for patients who have a weight lower than 30 kg.
Recent studies suggest that IL-1, produced by activated macrophages, plays a key part in eliciting CRS and ICANS. Anakinra, an IL-1 antagonist which is clinically available but not yet FDA approved for CART-associated toxicities, may lessen both CRS and ICANS after CART cell therapy [51,52]. Anakinra reduces the effect of IL-1α and IL-1β by competing for IL-1R binding and, in the clinic, 1–2 mg/kg/day is administered via subcutaneous injection [53]. Anakinra was able to effectively mitigate CRS and ICANS while maintaining CART cell antitumor efficacy in preclinical studies [50,53,54]. A recent clinical study showed promising results regarding the use of anakinra to alleviate CART-associated toxicities in large B-cell lymphoma [55]. Results from a phase I anti-CD22 CART cell trial showed that anakinra was associated with favorable outcomes when used in patients who developed hemophagocytic lymphohistiocytosis-like manifestations [56]. The ongoing clinical trials exploring the use of anakinra (NCT04432506, NCT04359784, NCT04148430, and NCT04205838), may show additional promising outcomes.
Granulocyte-macrophage colony-stimulating factor (GM-CSF) is produced by activated CART cells and stimulates myeloid cells, suggesting a role in CRS development. IL-6, a major cytokine implicated in CRS, is produced by tumor-resident myeloid cells, which are dependent on GM-CSF [57]. The Rosenberg group revealed that GM-CSF levels are highest during the initial phases of CRS [58]. Moreover, excess GM-CSF can cause ICANS and cerebral edema [59]. Initial GM-CSF surges are linked to more severe CRS [60]. A study conducted by Sterner et al. showed that neutralization of GM-CSF with lenzilumab or with CRISPR gene editing does not prevent antitumor functions of anti-CD19 CART cells in vitro or in vivo. In addition, effective control of leukemia and enhancement of CART cell proliferation was sustained in vivo after GM-CSF neutralization with lenzilumab. In an ALL patient-derived xenograft model of CRS and ICANS, neutralization of GM-CSF led to a decrease in myeloid and T cell infiltration in the central nervous system and a substantial reduction of neuroinflammation and CRS [61]. A study conducted by Sachdeva et al. [62] showed that GM-CSF neutralization with antibodies or with TALEN gene editing in CART cells eliminates macrophage production of CRS-associated cytokines, including IL-6, IL-8, and MCP-1. Anti-GM-CSF therapies have potential to reduce severe adverse effects and improve the safety profile of CART cell therapies while preserving anti-tumor activity.
7.2. Nonspecific Therapies: Steroids
In patients who do not respond to tocilizumab, steroids have been successfully used to alleviate CRS symptoms [50]. Corticosteroids are typically used as a second-line treatment due to potential reduction of CART cell persistence and antitumor efficacy [24,46]. To treat ICANS, corticosteroids are the first-line treatment because tocilizumab does not effectively cross the blood–brain barrier [63]. At present, a typical regimen for CRS comprises of 10 mg of IV dexamethasone administered every 6 h or 1 mg/kg of IV methylprednisolone administered every 12 h in cases of moderate to severe CRS [15]. Due to robust central nervous system infiltration, dexamethasone may be preferable to methylprednisolone. An obvious concern with using steroids in CART cell therapy is nonspecific immunosuppressive effects. However, in small doses and over short periods, corticosteroids continue to be useful for the management of CRS when combined with first-line treatments [64].
7.3. Endothelial Cell-directed Therapy: Defibrotide
Clinical trials have begun to evaluate the effectiveness of defibrotide in preventing ICANS in patients who are undergoing CART cell therapy for relapsed or refractory diffuse large B-cell lymphoma. Defibrotide was approved in the U.S and Europe as a treatment for adults and children who develop hepatic veno-occlusive disease. Researchers believe that defibrotide may prevent CART-associated ICANS by protecting endothelial cells from injury within the central nervous system. Currently, there are no published preclinical studies regarding the use of defibrotide to treat CART toxicities.
7.4. Small Molecule Inhibitors: JAK Inhibitors, Ibrutinib, Dasatinib
Small molecule inhibitors can interrupt signaling pathways which contribute to the onset of CRS. Ruxolitinib, an inhibitor of the Janus kinase (JAK)-signal transducer and activator of transcription (STAT) pathway, prevented the development of CRS while preserving anti-tumor efficacy and long-term survival in a mouse model of primary acute myeloid leukemia after treatment with CD123-specific CART cells [65]. JAK pathway inhibition by ruxolitinib disrupts IL-6 and GM-CSF signaling pathways to decrease CRS [50]. Another JAK/STAT pathway inhibitor, itacitinib, is selective for JAK1 and is being evaluated in a Phase 2 clinical trial for CRS management (NCT04071366).
Leukemic B cells can be targeted by Bruton’s tyrosine kinase (BTK) inhibitors to decrease abnormal cytokine production caused by aberrant BTK signaling. One BTK inhibitor, ibrutinib, is currently approved for relapsed CLL. Ibrutinib can reduce inflammatory cytokines such as IL-6, IFN-γ, and GM-CSF in a mouse model of CRS while preserving anti-CD19 CART cell activity [66]. Increased expression of CD200 in CLL diminishes the antitumor response of CD8+ T cells [67]. Fraietta et al. [68] showed that repeated administration of ibrutinib to CLL patients enhanced the proliferation of anti-CD19 CART cells and also reduced expression of CD200 on leukemic cells. In another study, CLL patients treated with CART cell therapy together with ibrutinib had lower severity of CRS and serum concentrations of cytokines associated with CRS while maintaining robust in vivo CART cell expansion [69,70]. Ibrutinib has the potential to prevent the development of CRS by decreasing key inflammatory cytokines and also improve CART cell activity through direct effects on leukemic cells.
Another tyrosine kinase inhibitor, dasatinib, was approved for the treatment of chronic myelogenous leukemia and Philadelphia chromosome-positive ALL. Dasatinib has additional functions such as inhibiting T cell signaling kinases and activation [71]. In two preclinical models, dasatinib showed reversible inhibition of anti-CD19 CART cell cytolytic activity, cytokine production, and proliferation [72,73]. Based on these studies, dasatinib is a promising strategy to prevent CART-associated CRS in a reversible and dose-dependent manner.
8. CONCLUSION
In summary, CART cell treatment has demonstrated promising clinical outcomes, but associated toxicities hinder the widespread use of this therapy. Further research and experience will shed new insight on toxicity prevention and management, removing barriers to the adoption of CART cell therapy as a front-line cancer treatment.
CONFLICTS OF INTEREST
SSK is an inventor on patents in the field of CAR immunotherapy that are licensed to Novartis (through an agreement between Mayo Clinic, University of Pennsylvania, and Novartis), Humanigen (through Mayo Clinic), and Mettaforge (through Mayo Clinic). SSK receives research funding from Kite, Gilead, Juno, Celgene, Novartis, Humanigen, Morphosys, Tolero, Sunesis, and Lentigen.
AUTHORS’ CONTRIBUTION
SK contributed in conception and design of the study. AZ contributed in literature search and acquisition of data. AZ and ES contributed in manuscript preparation. ES, SK and AZ contributed in critically revising the manuscript. SK contributed in mentor.
ACKNOWLEDGMENTS
This work was supported through grants K12CA090628 (SSK), the National Comprehensive Cancer Network (SSK), the Mayo Clinic Center for Individualized Medicine (SSK), and the Predolin Foundation (SSK).
REFERENCES
Cite this article
TY - JOUR AU - Anas Zahid AU - Elizabeth L. Siegler AU - Saad S. Kenderian PY - 2020 DA - 2020/11/23 TI - CART Cell Toxicities: New Insight into Mechanisms and Management JO - Clinical Hematology International SP - 149 EP - 155 VL - 2 IS - 4 SN - 2590-0048 UR - https://doi.org/10.2991/chi.k.201108.001 DO - 10.2991/chi.k.201108.001 ID - Zahid2020 ER -