Haploidentical Hematopoietic Stem Cell Transplantation Followed by ‘Post-Cyclophosphamide’: The Future of Allogeneic Stem Cell Transplant
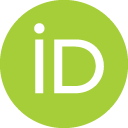
- DOI
- 10.2991/chi.d.200405.001How to use a DOI?
- Keywords
- Haploidentical; Allogeneic; Posttransplant cyclophosphamide
- Abstract
Allogeneic hematopoietic cell transplant (Allo-HCT) is a potentially curative therapy for many malignant and nonmalignant hematological diseases. However, a suitable human leukocyte antigens (HLAs)-matched donor may not be available when the patient is in urgent need of a stem cell transplant. This challenge has been ameliorated to a large extent by the introduction of haploidentical donors. This type of donor shares one HLA haplotype with the recipient. Therefore, a patient's full sibling has a 50% chance of being haploidentical and a patient's biologic parents and children will all be haploidentical, thus providing an immediately accessible, motivated donor for almost every recipient. Haploidentical transplants previously incurred prohibitively poor outcomes, preventing their widespread use. However, several recent advances have dramatically improved the results, making them a more viable donor source. In this review, we discuss different types of donors used for Allo-HCT with a particular focus on the use of haploidentical donors and their future potential.
- Copyright
- © 2020 International Academy for Clinical Hematology. Publishing services by Atlantis Press International B.V.
- Open Access
- This is an open access article distributed under the CC BY-NC 4.0 license (http://creativecommons.org/licenses/by-nc/4.0/).
1. INTRODUCTION
Allogeneic hematopoietic cell transplant (Allo-HCT) consists of cytotoxic conditioning, followed by transplantation of hematopoietic progenitor cells from a non-self-donor to reconstitute a patient's bone marrow. Allo-HCT has curative potential for a number of malignant and nonmalignant hematologic diseases. However, the difficulties in finding an appropriate donor have been a challenge since its inception in 1958.
2. DONOR TYPES
2.1. Matched-Related and Unrelated Donors
Human leukocyte antigens (HLAs) are cell surface proteins that enable the host's immune system to recognize and distinguish self from non-self-antigens. Class I and II HLA proteins play a critical role in the presentation of antigens to T cell receptors. Serologic and molecular typing of HLA genes allows matching between two individuals. The degree of immune disparity or similarity between the host and donor strongly affects the outcome of the transplant [1,2].
The most desirable donor is one who is fully matched, as this minimizes the allogeneic reactivity between the host and donor. However, only 30% of patients have a matched-related donor (MRD) [3,4]. Others must turn to bone marrow registries in pursuit of a matched-unrelated donor (MUD). Overall survival (OS) between MRD and MUD is similar; however, complications, most significantly graft vs. host disease (GvHD), are higher in the latter [5–7]. GvHD is the process by which the newly donated T cells (present in the graft) recognize the recipient (the host) as foreign and attack primarily the skin, gut and liver.
Although there are almost 35 million donors across 53 countries listed in the World Marrow Donor Association (WMDA), including nearly 9 million donors in the USA National Marrow Donor Program (NMDP), the likelihood of finding a 10/10 MUD varies greatly depending on a patient's racial and ethnic group [8]. White Europeans have a 75–80% chance of successfully identifying a match, while minorities have a significantly more difficult time finding a MUD. Hispanics successfully identify a match on the registry 34–65% of the time, South and Southeast Asians 27–60%, and African Americans 19–35% of the time [3,4,9]. This variation is due, in part, to underrepresentation of minorities in bone marrow registries. However, the disparities in identifying a successful match are out of proportion to their underrepresentation. These discrepancies are also a result of African and Asian Americans having a greater number of haplotypes when compared to White Europeans. This increased variability makes it less likely to find a suitable match for these groups [3,9].
Therefore, either because the severity of a patient's disease does not afford them the luxury of time, or because the uniqueness of their genetics does not dispose them to being easily matched, many patients must pursue alternative donor options: mismatched unrelated donor (MMUD), umbilical cord blood (UCB), or haploidentical donor.
2.2. Mismatched Unrelated Donor
A 7/8 or better MMUD is likely to be more closely matched than the alternative UCB or haploidentical options. With each additional HLA allele mismatch, OS at 5 years decreases by 10 percent [1,2]. HLA mismatch also correlates with an increased risk of GvHD [1,2]. Therefore, patients with MMUDs have historically had less GvHD compared to alternative unmatched options. However, newer studies directly comparing outcomes of haploidentical transplantations with MMUDs (as well as UCB and even with MRD and MUD) are challenging the standard of care [5,7,10–27].
MMUDs also pose problems for those patients requiring urgent transplants, as identifying a donor via a registry and coordinating a transplant takes an average of one to two and, in some studies, up to four months after initiating the search [28,29]. Even once a donor is identified, the attrition rate of an unrelated donor is 9–30% [30–32]. Patients are therefore often forced to seek alternative options.
3. UMBILICAL CORD BLOOD
Allo-HCT using UCB as a donor source has the advantages of an expanded donor pool, easy procurement, and removes the possibility of donor attrition, all of which accounts for a prompt availability with a time to transplantation averaging two to four weeks [4,33]. In addition, UCB transplants allow a greater degree of mismatching, likely due to immunologically naive T cells in cord blood, which incurs a lower risk of severe, acute GvHD for equivalent degrees of mismatch [34].
Disadvantages of UCB transplants include an increased risk of graft failure and delayed neutrophil and platelet engraftment, due to smaller numbers of hematopoietic progenitors compared with adult donors. The delay in immune reconstitution results in increased rates of infection [35–37]. Additionally, the source is limited—by its nature—to a one-time donation, and is unavailable for subsequent use should the patient relapse and require additional transplantations. Lastly, UCB also carries an increased risk of transmitting undetected hematologic diseases and autoimmune disorders [35–37].
Given the myriad issues with each of these sources, hematology has turned further investigation toward the use of haploidentical donors and realizing their potential.
4. HAPLOIDENTICAL DONOR
A haploidentical donor shares one HLA haplotype with the recipient. A patient's full sibling has a 50% chance of being haploidentical and a patient's biologic parents and children are all haploidentical to the patient, thus providing an immediately accessible, motivated donor for almost every recipient. The nearly universal availability of a haploidentical donor has made it an attractive option as a donor source for Allo-HCT and an active area of investigation.
Until recently, however, the degree of HLA mismatch, which leads to bidirectional alloreactivity, caused unacceptably high rates of GvHD and graft rejection, resulting in prohibitively poor outcomes [38–41]. In large part due to the advent of new conditioning and GvHD prophylactic regimens, such complications have been significantly reduced. In multiple recent studies of transplants for hematologic malignancies, haploidentical transplants had noninferior and, at times, superior outcomes compared with MMUDs, UCB, and even matched donors [5,7,10–27]. In this review, we will compare haploidentical donor outcomes with outcomes using other donor sources.
4.1. Haploidentical vs. UCB
A recent meta-analysis by Poonsombudlert et al. reviewed seven clinical trials that compared Allo-HCT using either haploidentical or cord blood donors in 3,434 patients and analyzed four outcome measures. Patients receiving haploidentical transplants had superior OS (pooled OR 1.71), lower rates of acute GvHD (pooled OR 0.78), and lower relapse rates (pooled OR 0.74). However, rates of chronic GvHD were higher (pooled OR 1.41) [26].
Other measures among a number of the individual trials within the meta-analysis frequently had noninferior, if not superior, outcomes for the haploidentical group, most notably across several survival points. Leukemia-free survival (LFS) and progression-free survival (PFS) were significantly greater in the haploidentical cohort in two of the studies [10,11] and notsignificantly different in four others [20–22,27]. Nonrelapse mortality (NRM) was significantly better in two of the investigations [10,21] and was not significantly different in five others [11,20,22,23,27].
The risk of infection-related mortality, a concern particular to UCB transplants because of delayed immune reconstitution and longer time to engraftment, and also historically associated with haploidentical transplants, was inconsistent among the studies within the meta-analysis [26]. One trial showed lower rates of infection-related mortality in the haplo group (25% vs. 35%) [10], one demonstrated no difference (30% vs. 30%) [22], and a third reported lower rates in the UCB group (68% vs. 56%) [21].
The decreased incidence of GvHD in recipients of haploidentical transplants, particularly acute GvHD, which has a stronger correlation with mortality than does chronic GvHD, likely accounts for the superior survival outcomes. The higher rates of chronic GvHD and corresponding lower relapse rates highlight the importance of the graft vs. leukemia (GVL) effect. Just as donor T cells attack the host in GvHD, they also attack leukemic cells, preventing relapse and extending LFS.
4.2. Haploidentical vs. MMUD
Haploidentical transplantations have recently been shown to have equivalent outcomes when compared to MMUD transplants. In a multicenter retrospective analysis of patients transplanted for acute leukemia, Piemontese et al. compared outcomes by donor source: haploidentical, MUD, MMUD [16]. There was no difference in three-year OS (46% vs. 48%), LFS (41% vs. 46%), NRM (29% vs. 29%), and relapse incidence (30% vs. 25%) in haploidentical compared to 9/10 MMUD transplants. Also of note, the incidences of acute and chronic GvHD were similar in both groups (28% vs. 27% and 34% vs. 33%, respectively) [16].
A Phase 2 trial of allo-HCT for high-risk malignancies compared the use of posttransplant cyclophosphamide (PTCy) in patients undergoing haploidentical or MMUD transplants and found similar results in all survival and GvHD-related measures. There was no difference in two-year OS (55% vs. 52%), PFS (53% vs. 42%), NRM (23% vs. 34%), or relapse incidence (24% vs. 25%). The cumulative incidence of acute and chronic GvHD incidences (33% vs. 40% and 24% vs. 19%, respectively) were also similar [12]. A third study by Baker et al. investigated patients transplanted for all hematologic malignancies and had nearly identical results, demonstrating similar outcomes in OS, PFS, relapse rates, as well as cumulative acute and chronic GvHD [13].
Even when transplanted for a different indication, these results were reproducible. An Allo-HCT trial for Hodgkin lymphoma (HL) by Gauthier et al. reported similar OS, event-free survival, and NRM in haploidentical donors and MMUDs [17]. In that study, however, patients in the haploidentical cohort had significantly lower rates of severe, acute GvHD (3% vs. 9%), and chronic GvHD (15% vs. 48%). This resulted in a superior three-year GvHD-free relapse-free survival (GRFS) (52% vs. 31%) [17].
These data demonstrate consistently comparable survival measures between the two donor sources, as well as largely similar incidences of GvHD, with the exception of the Gauthier study, which observed lower rates in the haploidentical donor group.
HLA-DPB1 permissive mismatching is another factor pertinent to MMUD selection. T-cell epitope groups may be assigned to recipients and unrelated donors based on cross-reactivity patterns [42,43]. Those with minimal responses are classified as permissive HLA-DPB1 mismatch, and those T cells which generate strong responses are classified as nonpermissive mismatches [42,43]. Predictably, nonpermissive DPB1 mismatches were associated with higher rates of severe, acute GVHD, and NRM when compared to matched DPB1 or permissive DPB1 mismatches [42,43]. One study also demonstrated significantly worse overall mortality in the nonpermissive DPB1 mismatch group [42] while a second had a nonsignificant trend toward inferior mortality [43]. Of note, permissive DPB1 mismatches did not have significantly worsen acute GvHD or OS when compared to DPB1 matches, though they did have inferior NRM [42]. Accordingly, it is essential to try to find a matched, or at least permissive mismatch, HLA-DPB1 donor in the case of MUDs. However, given the HLA mismatches between donor and recipient in haploidentical transplants, the HLA-DPB1 status plays no role.
4.3. Haploidentical vs. MRD and MUD
Given the successful outcomes of Allo-HCT from haploidentical donors compared with that from mismatched donor sources, interest has turned to comparing this group with matched related and unrelated donors. A meta-analysis by Gu et al. of nine case-control studies including a total of 2,258 patients compared outcomes among haploidentical, MRD, and MUD sources [7]. There were no significant differences in OS (RR 0.93 [0.83–1.04]), PFS (RR 1.06 [0.93–1.22]), NRM (RR 0.99 [0.73–1.35]), or relapse rates (RR 1.01 [0.88–1.16]) between the haploidentical donor and MRD groups, nor was there a difference between the haploidentical group and MUD group in OS (RR 1.04 [0.90–1.20]), PFS (RR 1.19 [0.95–1.49]), NRM (RR 0.83 [0.62–1.09]), or relapse rates (RR 0.96 [0.77–1.21]) [7].
Most notably, there was no difference in acute GvHD between the haploidentical donor and the MRD (RR 1.13 [0.63–2.02]) or the MUD groups (RR 0.94 [0.78–1.13]), as well as severe, acute GvHD (RR 0.98 [0.52–1.83] and 0.95 [0.68–1.32], respectively), historic points of superiority for matched donor transplantation. Furthermore, rates of chronic GvHD in the haploidentical group were lower than in MRD, with RR 0.54 [0.39–0.75], and also lower than in the MUD cohort (RR 0.70 [0.56–0.88]) [7]. This is especially remarkable given that the degree of donor HLA mismatch has long been correlated with the development of GvHD [1,2].
Additional trials have confirmed the findings in this meta-analysis showing similar survival and GvHD outcomes for Allo-HCT using haploidentical or MRD and MUD. A study by Lorentino et al. compared haploidentical donors with 10/10 MUD and 9/10 MMUDs in those transplanted for acute myeloid leukemia (AML) [14]. All three groups had nonsignificantly different two-year OS (59% vs. 50% vs. 50%), LFS (53% vs. 43% vs. 44%), NRM (19% vs. 18% vs. 18%), or relapse rates (27% vs. 39% vs. 33%). Furthermore, rates of acute and chronic GvHD were similar, a dramatic difference compared to previous trials between these donor types [14].
Transplants for other indications have demonstrated similar results. In patients transplanted for HL and Philadelphia-chromosome positive acute lymphocytic leukemia (ALL), OS, PFS, and acute GvHD were similar in haploidentical transplants compared to MUD transplants [6,19]. In the HL study, NRM (17% vs. 21%) and chronic GvHD (34% vs. 40%) were significantly lower in the haploidentical donor group [6].
Importantly, these results were found to be similar in patients over 60 years of age undergoing Allo-HCT. Santoro et al. and Devillier et al. evaluated patients in this age group with AML who received transplants from either haploidentical donors or MUDs [5,24]. Neither study showed any difference in OS, LFS, or NRM [5,24]. The Devillier study also reported significantly higher rates of acute and chronic GvHD in the MUD group than in the haploidentical donor group [24].
Though most trials comparing outcomes for haploidentical donors to MRDs observed similar survival outcomes, the incidence of GvHD continues to be lower in MRDs. This was the case in two such investigations, which analyzed patients transplanted for HL as well as HL and non-Hodgkin lymphoma (NHL) [6,18]. When comparing haploidentical donors to MRDs, there was no significant difference in rates of two-year and three-year OS (67% vs. 71% and 61% vs. 62%, respectively), PFS (43% vs. 45% and 48% vs. 48%, respectively), and NRM (17% vs. 21% and 15% vs. 13%, respectively) [6,18]. The trial which analyzed only HL patients did find significantly more acute GvHD (33% vs. 18%) in the haploidentical cohort [6], while the study which analyzed HL and NHL patients had equal incidences of acute GvHD and, surprisingly, lower rates of chronic GvHD in the haploidentical group [18].
Even for patients with high-risk malignancies, survival outcomes appear to be similar with either haploidentical or MRD transplant. A retrospective study by Liu et al. comparing haploidentical transplants with matched sibling donors (MSD) for AML patients with high-risk cytogenetics showed similar rates of OS, LFS, transplant-related mortality (TRM), and relapse [15]. Of note, the haploidentical group did have significantly worse rates of acute GvHD (70.9% vs. 30.2%) and a nonsignificant trend toward higher incidences of severe acute GvHD (15% vs. 7%) [15].
A second retrospective study by Salvatore et al. stratified outcomes by cytogenetics. Across all patients studied with AML, MSDs had superior OS, LFS, NRM, GvHD, and engraftment rates [25]. However, among patients with high-risk cytogenetics, the rates of two-year OS (67% vs. 66%), LFS (61% vs. 55%), and NRM (18% vs. 10%) were similar. The incidence of acute GvHD was worse in the haploidentical cohort (36% vs. 24%), though relapse rates were lower (21% vs. 36%) [25].
These studies, with consistently worse rates of GvHD, comparable survival outcomes, and superior relapse rates in patients transplanted for high-risk disease with haploidentical stem cells, again reflect the importance of GVL. Though this pathophysiology is present in intermediate and low-risk malignancies, its subsequent effect on survival and relapse appears to yield greater clinical significance in high-risk situations. Table 1 summarize the studies comparing haploidentical stem cell transplants with the other various types of allogeneic stem cell transplants including MUD, MSD, MMUD and umbilical cord blood transplants.
First Author [Citation Number] | Article Type | Donor Types | Transplant Indication | Number of Patients | Analysis Type | OS | LFS/PFS | NRM | RR | aGvHD | cGvHD |
---|---|---|---|---|---|---|---|---|---|---|---|
Poonsombudlert [26] | Meta-analysis | Haplo vs. UCB | Various | 3434 | Pooled Odds Ratio | 1.77 [1.1–2.87] | – | – | 0.74 [0.57–0.97] | 0.78 [0.67–0.92] | 1.41 [1.02–1.95] |
Giannotti [10] | Retrospective study | Haplo vs. UCB | AML | 333 | Univariate Analysis | 69% vs. 42% (p < 0.001) | 63% vs. 40% (p < 0.001) | 21% vs. 48% (p < 0.001) | 17% vs. 12% (p = 0.709) | 26% vs. 29% (p = 0.853) | 33% vs. 37% (p = 0.492) |
El-Cheikh [11] | Retrospective Study | Haplo vs. UCB | Various | 150 | Univariate Analysis | 69% vs. 45% (p = 0.10) | 65% vs. 36% (p = 0.01) | 18% vs. 23% (p = 0.49) | 18% vs. 38% (p = 0.03) | 34% vs. 50% (p = 0.08) | 6% vs. 12% (p = 0.001) |
Kanate [27] | Retrospective Study | Haplo vs. UCB | Various | 86 | Univariate Analysis | 78% vs. 70% (p = 0.48) | 65% vs. 62% (p = 0.75) | 14% vs. 19% (p = 0.59) | 20% vs. 19% (p = 0.90) | 16% vs. 16% (p = 0.99) | – |
Ruggeri [22] | Retrospective Study | Haplo vs. UCB | Acute Leukemias | 1446 | Hazard Ratio Univariate analysis (for a GvHD only) |
– | AML: 1.0 vs. 0.78 [0.84–1.25] ALL: 1.0 vs. 1.002 [0.79–1.31] |
AML: 1.0 vs. 1.16 [0.82–1.50] ALL: 1.0 vs. 1.23 [0.87–1.75] |
AML: 1.0 vs. 0.95 [0.73–1.25] ALL: 1.0 vs. 0.82 [0.57–1.19] |
AML: 27% vs. 31% (p = 0.10) ALL: 31% vs. 37% (p = 0.71) |
AML: 1.0 vs. 0.63 [0.45–0.88] ALL: 1.0 vs. 0.58 [0.38–0.88] |
Robin [21] | Retrospective Study | Haplo vs. MMUD vs. UCB | MDS | 833 | Hazard Ratio | 1.0 vs. 1.32 [1.03–1.60] vs. 1.65 [1.23–2.23] | 1.0 vs. 1.29 [1.02–1.62] vs. 1.51 [1.14–2.02] | 1.0 vs. 1.29 [0.96–1.74] vs. 1.68 [1.17–2.41] | 1.0 vs. 1.27 [0.88–1.83] vs. 1.25 [0.78–2.01] | 1.0 vs. 1.68 [1.13–2.49] vs. 1.52 [0.93–2.49] | 1.0 vs. 1.17 [0.85–1.60] vs. 1.07 [0.72–1.61] |
Gauthier [17] | Retrospective Study | Haplo vs. MMUD vs. UCB | Hodgkin Lymphoma | 98 | Univariate Analysis | 75% vs. 73% vs. 80% (p = 0.57) | – | 9% vs. 11% vs. 18% (p = 0.45) | 25% vs. 25% vs. 36% (p = 0.52) | 28% vs. 27% vs. 45% (P = 0.016) | 15% vs. 48% vs. 49% (p = 0.006) |
Baron [20] | Retrospective Study | Haplo vs. MSD vs. MUD vs. UCB | AML | 1715 | Univariate Analysis | 43% vs. 59% vs. 53% vs. 56% (p = 0.02) | 44% vs. 54% vs. 50% vs. 50% (p = 0.014) | 22% vs. 13% vs. 20% vs. 16% (p < 0.001) | 34% vs. 32% vs. 30% vs. 34% (p = 0.70) | 31% vs. 19% vs. 30% vs. 38% (p < 0.001) | 30% vs. 50% vs. 51% vs. 28% (p < 0.001) |
Piemontese [16] | Retrospective Study | Haplo vs. MUD Haplo vs. MMUD |
Acute Leukemias | 3568 | Hazard Ratio | 1.0 vs. 0.71 [0.58–0.86] 1.0 vs. 0.93 [0.72–1.18] |
1.0 vs. 0.75 [0.62–0.90] 1.0 vs. 0.92 [0.72–1.15] |
1.0 vs. 0.64 [0.48–0.84] 1.0 vs. 0.99 [0.78–1.40] |
1.0 vs. 0.86 [0.67–1.15] 1.0 vs. 0.84 [0.63–1.13] |
1.0 vs. 0.89 [0.68–1.16] 1.0 vs. 1.04 [0.77–1.41] |
1.0 vs. 1.08 [0.85–1.37] 1.0 vs. 0.96 [0.73–1.16] |
Baker [13] | Retrospective Study | Haplo vs. URD | Various | 113 | Univariate Analysis | 465d vs. 403d (p = 0.969) | 245d vs. 293d (p = 0.666) | – | 44% vs. 49% (p = 0.642) | 63% vs. 53% (p = 0.269) | 22% vs. 20% (p = 0.165) |
Gu, Z [7] | Meta-analysis | Haplo vs. MRD | Various | 2258 | Risk Ratio | 0.93 [0.83–1.04] | 1.06 [0.93–1.22] | 0.99 [0.73–1.35] | 1.01 [0.88–1.16] | 1.13 [0.63–2.02] | 0.54 [0.39–0.75] |
Haplo vs. MMUD | 1.04 [0.90–1.02] | 1.19 [0.95–1.49] | 0.83 [0.62–1.09] | 0.96 [0.77–1.21] | 0.94 [0.78–1.13] | 0.7 [0.56–0.88] | |||||
Lorentino [14] | Retrospective Study | Haplo vs. MUD vs. MMUD |
Adverse karyotype AML | 630 | Hazard Ratio | 1.0 vs. 1.20 [0.77–1.87] vs. 1.24 [0.76–2.03] | 1.0 vs. 1.22 [0.80–1.86] vs. 1.28 [0.80–2.06] | 1.0 vs. 0.93 [0.48–1.81] vs. 0.99 [0.47–2.14] | 1.0 vs 1.45 [0.83–2.52] vs. 1.51 [0.81–2.79] | 1.0 vs. 1.11 [0.67–1.87] vs. 1.32 [0.74–2.35] | 1.0 vs 1.21 [0.68–2.14] vs. 1.33 [0.70–2.53] |
Gu, B [19] | Retrospective Study | Haplo vs. MUD | Ph+ ALL | 58 | Univariate Analysis | 69% vs. 67% (p = 0.638) | 64% vs. 63% (p = 0.708) | 14% vs. 19% (p = 0.869) | 19% vs. 6% (p = 0.466) | 43% vs. 47% (p = 0.517) | 19% vs. 13% (p = 0.637) |
Santoro [5] | Retrospective Study | Haplo vs. MUD | AML >60 yo | 2839 | Univariate Analysis | 39% vs. 42% (p = 0.33) | 35% vs. 40% (p = 0.67) | 38% vs. 28% (p = 0.06) | 28% vs. 32% (p = 0.17) | 31% vs. 33% (p = 0.28) | 27% vs. 41% (p = 0.24) |
Martinez [6] | Retrospective Atudy | Haplo vs. MSD Haplo vs. MUD |
Hodgkin Lymphoma | 709 | Univariate Analysis | 67% vs. 71% (p = 0.51) 67% vs. 62% (p = 0.44) |
43% vs. 38% (p = 0.8) 43% vs. 45% (p = 0.91) |
17% vs. 13% (p = 0.37) 17% vs. 21% (p = 0.30) |
39% vs. 49% (p = 0.039) 39% vs. 32% (p = 0.31) |
33% vs. 18% (p = 0.003) 33% vs. 30% (p = 0.36) |
26% vs. 25% (p = 0.90) 26% vs. 41% (p = 0.04) |
Ghosh [18] | Retrospective Atudy | Haplo vs. MSD | Hodgkin and Non-Hodgkin Lymphomas | 987 | Univariate Analysis | 61% vs. 62% (p = 0.82) | 48% vs. 48% (p = 0.96) | 15% vs. 13% (p = 0.41) | 37% vs. 40% (p = 0.51) | 27% vs. 25% (p = 0.84) | 12% vs. 45% (p < 0.001) |
Liu [15] | Retrospective Study | Haplo vs. MSD | High-risk AML | 170 | Univariate Analysis | 64% vs. 63% (p = 0.565) | 63% vs. 52% (p = 0.462) | – | 22% vs. 30% (p = 0.482) | 44% vs. 28% (p = 0.061) | – |
Salvatore [25] | Retrospective Study | Haplo vs. MSD | Intermediate or High Risk Cytogenetics AML | 2654 | Univariate Analysis | 68% vs. 76% (p < 0.001) | 58% vs. 67% (p < 0.001) | 23% vs. 10% (p < 0.01) | 19% vs. 24% (p = 0.10) | 31% vs. 21% (p < 0.01) | 35% vs. 33% (p = 0.05) |
Haploidentical Stem Cell Transplants Compared to MUD, MSD, MMUD and Umbilical Cord Blood Transplants
5. PTCy: PREVENTING GVHD
Haploidentical transplants have previously been associated with unacceptably high rates of GvHD, limiting the utility of this donor source [38–41]. The strategy of using PTCy as an adjunct to current GvHD prophylaxis regimens has markedly improved haploidentical transplant outcomes, enabling this donor source to be a viable option [44–47].
When used for GvHD prophylaxis in haploidentical transplantation, cyclophosphamide is administered prior to transplant and then on days 3+ and 4+ following stem cell infusion [44]. It is typically given in combination with mycophenalate mofetil (MMF) and calcineurin inhibitors (cyclosporine or tacrolimus), as well [44].
Cyclophosphamide is metabolized by the hepatic CYP450 enzyme into its active metabolites, which exist in equilibrium with aldophosphamide. Aldophosphamide is then either oxidized by aldehyde dehydrogenase (ALDH) or diffuses into cells and is decomposed into phosphoramide mustard where it forms DNA crosslinks and induces apoptosis. Cells with increased ALDH expression are therefore disproportionately spared as they predominantly oxidize aldophosphamide, shunting it away from the apoptotic pathway. Proliferating alloreactive donor T cells, the primary mediators of GvHD, have decreased levels of ALDH and are preferentially killed. Regulatory T cells, mediators in the amelioration of GvHD, and stem cells have increased ALDH expression, and are spared [48–51].
The efficacy of PTCy in preventing GvHD has been demonstrated in several studies [44–47]. Ruggeri et al. compared it to anti-thymocyte globulin (ATG) for GvHD prophylaxis in patients with AML. Patients receiving PTCy had significantly reduced rates of severe, acute GvHD (5% vs. 12%) and, subsequently, higher rates of two-year GvHD-free survival (51% vs. 39%) and OS (58% vs. 54%). Interestingly, despite the association of GvHD with a GVL effect, LFS was also superior in the PTCy group (56% vs. 47%) [44].
Other observational studies and prospective trials analyzing patients transplanted for hematologic malignancies demonstrated similar outcomes with two-year OS rates of 44–77%, severe, acute GvHD as low as 4–8%, chronic GvHD incidences of 20–36%, and NRM rates of 10–20% [45–47].
6. COMPLICATIONS OF HAPLOIDENTICAL TRANSPLANT
6.1. Haplo-Immunostorm
As the use of haploidentical donor transplants increases, the recognition and appropriate management of serious associated complications is critical. Haplo-immunostorm (HIS), a syndrome similar to cytokine release syndrome (CRS), is a florid cytokine release induced by antibody–antigen interactions resulting in a systemic inflammatory cascade leading to fevers, tachycardia, vasodilatory shock, and respiratory failure. A range of severity from grade 1 to 4 has been outlined based on the degree of fever, hypotension, hypoxia, and end-organ damage, specifically hepatic involvement [52,53].
A majority of patients develop some degree of CRS (73–87%) and a significant minority (12–18%) meet the criteria for severe CRS (grade 3–4) [54,55]. However, this distinction is critical, as the cohort of patients who develop severe CRS have significantly worse outcomes when compared to patients with only mild CRS (grade 1–2). This includes worse median survival (2.6 months vs. 13.1 months), two-year OS (21% vs. 53%), and NRM (20% vs. 78%) [22]. Outcomes following mild CRS are similar to those of patients without CRS [54].
The shorter survival in patients with severe CRS may be a consequence of delayed neutrophil engraftment, as those patients with severe CRS took an average of 10 days longer to engraft when compared to patients with mild or no CRS (29 days vs. 16 days vs. 18 days, respectively). This delay subsequently leaves patients at increased vulnerability to infection [54].
Interestingly, in two studies which compared haploidentical donor grafts collected from peripheral blood or bone marrow, severe CRS only occurred in patients receiving peripheral blood stem cells [54,55]. The difference may be due to the fact that the latter contains a larger number of T cells, which may be involved in triggering the inflammatory cascade. CRS has a known association with an increase in interleukin levels, particularly IL-6. It is on this basis that treatment is targeted.
Tocilizumab, an anti-IL-6 receptor monoclonal antibody is an effective therapy in patients with CRS, leading to clinical resolution of CRS within an average of 48 hours. A mortality benefit has not yet been demonstrated though, as the major effect appears to be in those patients with mild CRS only [54]. It is unclear what predisposes certain patients to develop severe CRS. The identification of these risk factors and contributors could aid in guiding treatment.
6.2. Infection
The use and extent of immunosuppression following Allo-HCT is a hallmark of transplant medicine. The benefits of preventing graft rejection and GvHD must be balanced with the risks of infection. Despite appropriate prophylactic measures, infection is among the leading causes of morbidity and mortality after allogeneic transplantation [56,57]. Among haploidentical transplants, viral and fungal infections are the most common causes of morbidity and mortality [58]. Overall, higher rates of mismatch are associated with an increase in infectious complications [58]. However, the recent advances in conditioning regimens and immunosuppression have led to substantial improvements in infection rates in haploidentical transplants.
In patients who underwent Allo-HCT for AML the incidence of fatal infections among haploidentical transplantations (11%) was lower than among both MUDs (14%) and UCB (17%), but remained higher than in MSDs (4%), which require less immunosuppression [59]. Similar patterns have been demonstrated for fungal infections (haploidentical—11% vs. MSDs—4% vs. MUDs—14% vs. UCB—17%) as well as bacterial infections (haploidentical—25% vs. MSDs—23% vs. MUDs—36% vs. UCB—39%) [59]. The risk of bacterial infections is highest within the first 30 days after transplant. The most important factor in determining this risk is the time to neutrophil recovery. As a result of improved engraftment rates, bacterial infections in haploidentical transplants are no longer inferior to alternative donor sources [59].
Viral infections and its related mortality, on the other hand, are higher in haploidentical transplant, with a viral infection incidence of 70% at 100 days and 77% at one year. Cytomegalovirus (CMV) is the most commonly encountered viral infection, though Herpes simplex virus (HSV), Epstein–Barr virus (EBV)—a common cause of posttransplant lymphoproliferative disorder—varicella zoster, influenza, respiratory syncytial virus, adenovirus, parvovirus, and JC virus are also well described. Given the high rates of complications, acyclovir prophylaxis for HSV is the standard of care. Ganciclovir and foscarnet for CMV have severe complications (neutropenia and renal toxicity, respectively) which outweigh their benefits when used for prophylaxis, but are still indicated for treatment [58].
Fungal infections are also common causes of morbidity in this population. They most frequently occur early in the posttransplant time course with Aspergillus and Candida being the most common pathogens, though mucormycosis and fusarium are also not infrequent. Fluconazole prophylaxis has demonstrated efficacious risk reduction of invasive fungal infections, mainly because of its activity against Candida. It is ineffective against molds, specifically Aspergillus, which requires posaconazole or itraconazole and, accordingly, posaconazole had been the drug of choice for anti-fungal prevention in patients undergoing haploidentical stem cell transplant, given the prolong immunosuppression these patients endure [58,59].
6.3. Graft vs. Host Disease
Prior to the use of PTCy, GvHD was a common complication of haploidentical transplant, contributing to prohibitively poor outcomes [38–41]. In addition to the inclusion of PTCy, standard GvHD prophylaxis is based upon immunosuppression, primarily with calcineurin inhibitors, such as cyclosporine or tacrolimus, with or without methotrexate [60,61]. Treatment of GvHD requires more aggressive immunosuppression, initially with corticosteroids and in steroid-refractory patients, with the addition of other immunosuppressive agents such as mycophenolate mofetil (MMF) [62]. Though there still remains a great deal of room for improvement, the recent advances in GvHD prophylaxis account for a significant portion of the rise in the use of haploidentical donors.
7. DONOR SELECTION FROM AMONG HAPLOTYPES
Since a single patient may have multiple haploidentical donors, other factors should be considered in deciding which donor will be most suitable. These factors include gender mismatch, killer cell immunoglobulin-like receptor (KIR) ligands, donor specific antibodies (DSAs), and CMV serostatus.
7.1. Gender Mismatch
A female donor for a male recipient is a known poor prognostic factor, due to immune female T cells reacting to the genes present on the Y chromosome. It results in higher rates of GvHD as well as worse NRM, LFS, and OS [63,64]. A recent study by Gorin et al. compared female MSDs for male recipients with haploidentical donors for patients being treated for AML. In intermediate-risk AML, the outcomes were mixed. The haploidentical donor group had less chronic GvHD; the female MSD group had better LFS and OS. GRFS survival was comparable. However, in high-risk AML patients, haploidentical recipients had lower rates of relapse as well as superior GRFS, LFS, and OS [65].
In addition to donor gender, a haploidentical donor will either be maternally or paternally HLA matched, with the other being the mismatched haplotype. As a fetus, the recipient was exposed to maternal cells and therefore demonstrates a hypo-responsiveness to noninherited maternal antigens. Consequently, mismatched maternal haploidentical donors have been found to be better tolerated by the patient with lower rates of acute GVHD when compared to a mismatched paternal haploidentical donor, with all other variables being equal [66]. There are not currently any studies comparing maternally mismatched haploidentical donors to MSDs.
7.2. Killer Cell Immunoglobulin KIR Ligands
KIR ligands are another haplotype that can help distinguish haploidentical donors. KIR haplotypes can be inhibitory (KIR A) or activating (KIR B). Since natural killer cells aid in the GVL effect without GvHD, those with KIR B have better survival and decreased rates of relapse compared to those with KIR A [67]. However, data regarding KIR-ligand mismatching are inconsistent. Wanquet et al. demonstrated that patients with donors who possessed mismatched KIR-ligands had lower rates of relapse and longer periods of PFS, while Shimoni et al. identified a trend toward higher rates of relapse and inferior OS. Neither was associated with significantly different incidences of GvHD [68,69].
7.3. Donor-Specific Antibodies
DSAs bind to HLA and non-HLA molecules expressed on the graft, inducing complement-dependent mechanisms that recruit natural killer cells, which promote graft rejection. In solid organ transplant, they have been associated with increased rejection and decreased survival [70,71]. In haploidentical transplant, the presence of a DSA was found to be associated with a graft failure rate of 75% compared to 5% in those patients without DSA [72]. Two other studies corroborated these findings with strong associations between the presence of DSA and increased incidence of primary graft failure, TRM, and OS [73,74]. Therefore patients with DSAs should be desensitized to the donor before transplant and the desensitization should include plasmapheresis and Rituximab.
7.4. CMV Serostatus
CMV is responsible for substantial morbidity and mortality following Allo-HCT. The primary driver, though, is the serostatus of the patient, not the donor. Among haploidentical transplants, CMV+ recipients using CMV- donors compared to CMV+ donors had no difference in NRM or OS [75,76]. This remained true for CMV- recipients. However, recipients who were CMV+ had higher NRM and worse OS compared to those who were CMV-. These outcomes were consistent, regardless of the serostatus of the donor [75].
8. CONCLUSION
Allo-HCT remains the only curative therapy for multiple malignant and nonmalignant diseases, but identifying a suitable donor presents a challenge. Recent advances in haploidentical transplants, namely the advent of PTCy, have had a substantial influence in reducing complications and improving survival, making haploidentical donors not merely a feasible alternative but, at times, a preferable one. When compared to UCB transplants, haploidentical ones had decreased rates of acute GvHD and, at times, superior survival outcomes. Compared to MMUDs and MUDs, haploidentical donors offered consistently comparable survival measures, and frequently demonstrated similar incidences of GvHD. The data for MRDs are more variable. MRDs continue to be a preferred donor source, though a number of studies did identify nonsignificantly different survival measures, particularly in Allo-HCT for patients with high-risk disease. These results may be due to the increased importance of GVL in aggressive malignancies. The role of GVL in these situations highlights the potential of what could be accomplished if one is able to harness the GVL response while simultaneously suppressing GvHD.
The newfound success of haploidentical transplantation is due in large part to the effectiveness of PTCy in reducing the incidence of GvHD. However, its mechanism is not specific to the nature of the haploidentical donor, and as we learn about its utility in other donor groups, it has the potential to revolutionize GvHD prevention in the near future.
Furthermore, as transplant medicine becomes increasingly personalized and treatment is tailored to disease subtype and to specific donor and recipient subgroups, there may be certain circumstances in which a haploidentical donor proves to be the primary preferred choice, even compared to a MSD alternative. Yet, as the majority of the studies discussed in our review article are retrospective, further investigation is warranted to truly evaluate the great potentials of haploidentical donors. Nevertheless, there is a clear and strong implication that haploidentical transplants and PTCy are going to play a large role in the future of allogeneic stem cell transplants.
CONFLICT OF INTEREST
No conflicts of interest relevant for this paper from both authors.
AUTHORS' CONTRIBUTIONS
S.C. and M.A.H. both had equal contribution to the conception of the work, drafting the work, revising it and approval of the final manuscript.
Funding Statement
No funding was received for this study.
REFERENCES
Cite this article
TY - JOUR AU - Samuel Cytryn AU - Maher Abdul-Hay PY - 2020 DA - 2020/04/19 TI - Haploidentical Hematopoietic Stem Cell Transplantation Followed by ‘Post-Cyclophosphamide’: The Future of Allogeneic Stem Cell Transplant JO - Clinical Hematology International SP - 49 EP - 58 VL - 2 IS - 2 SN - 2590-0048 UR - https://doi.org/10.2991/chi.d.200405.001 DO - 10.2991/chi.d.200405.001 ID - Cytryn2020 ER -