Hepatic arterial changes following iodized oil chemoembolization of hepatocellular carcinoma: Incidence and technical consequence
- DOI
- 10.1016/j.artres.2011.08.002How to use a DOI?
- Keywords
- Hepatic artery; Iodized oil; Chemoembolization; Hepatocellular carcinoma
- Abstract
Objective: To describe the nature, incidence, and therapeutic consequence of hepatic arterial changes seen following transcatheter arterial chemoembolization (TACE) for hepatocellular carcinoma (HCC).
Methods: In this retrospective study, 46 patients with HCC underwent ≥ 2 TACE treatment sessions between 2004 and 2010. All patients had hepatic angiography on days of treatment. Sequential angiographic studies were reviewed to assess for abnormalities in appearance of the hepatic vasculature. Angiographic abnormalities were graded in a binary fashion: present or absent. When present, abnormalities and effect on drug delivery were recorded.
Results: 123 (mean 2.7, range 2–5) successful lobar (n = 34), segmental (n = 88), or superselective (n = 1) TACE procedures were performed in 46 patients (M:F = 36:10, mean age 59 years). TACE was performed using 1:1 chemotherapy to iodized oil mixture without (n = 102) or with (n = 21) particle embolization. An abnormal angiographic appearance was identified in 21/46 (38%) patients and in 23/123 (19%) procedures, with first appearance after mean 1.5 (range 1–3) TACE sessions and mean 176 (range 27–509) days after initial TACE. Abnormalities included new vessel attenuation or stenosis (n = 10, 43%), slow flow (n = 2, 9%), and new vascular occlusions (n = 11, 48%). These vascular changes did not result in inability to perform repeat TACE in 16/16 (100%) cases where vascular changes were present and TACE was repeated to the same liver lobe.
Conclusion: While the hepatic vasculature is altered in many patients undergoing TACE, arterial abnormalities did not preclude therapy.Further investigation is warranted.
- Copyright
- © 2011 Association for Research into Arterial Structure and Physiology. Published by Elsevier B.V. All rights reserved.
- Open Access
- This is an open access article distributed under the CC BY-NC license.
Introduction
Transcatheter arterial chemoembolization (TACE) is an established liver directed intra-arterial treatment for hepatocellular carcinoma (HCC) which confers survival benefit to patients with surgically unresectable tumors.1,2 This therapy exploits the discrepancy in blood supply between the hypervascular neoplastic tissue of HCC, which derives 90–100% of its blood flow from the hepatic artery, and non-tumorous liver parenchyma, which is predominantly perfused by the portal vein.3 A combination of chemotherapeutic drugs and embolic agents are delivered via the hepatic artery directly to the tumor, resulting in local tumor devascularization and chemotherapy drug retention.4,5 Because tumor response rates following TACE range from only 31–64% and time to disease progression averages 7.9 months,6 patients with HCC often require repeated TACE therapy to treat residual, recurrent, or newly developed tumor. However, the caustic nature of chemotherapeutic agents administered during TACE may precipitate injury to the hepatic artery system,7 potentially resulting in elimination of future arterial access to tumor and limitation of TACE therapy. Hepatic arterial damage has been reported to occur in 8–48% of patients, with loss of vascular patency in 56–81% of cases when TACE is performed to a stasis or near stasis angiographic endpoint.8–11 This study was undertaken to assess the nature and incidence of hepatic arterial changes seen following iodized oil TACE for treatment of HCC when performed to a substasis angiographic endpoint, and to evaluate the degree of therapeutic limitation on future TACE resulting from hepatic arterial damage.
Materials and methods
This retrospective study was approved by our hospital’s Institutional Review Board, and was in compliance with the Health Insurance Portability and Accountability Act. All patients provided written informed consent for procedures.
Clinical setting, patients, and tumors
Between January 2004 and March 2010, 46 patients with surgically unresectable HCC who underwent a total of 123 TACE procedures were identified among all patients undergoing TACE at a single academic university affiliated hospital situated in a large metropolitan area and were selected for study. Inclusion criteria for TACE consisted of (a) age greater than 18 years, (b) surgically unresectable HCC, (c) ability to undergo angiography and selective visceral catheterization, (d) ability to lay supine greater than 30 min, (e) ability to provide informed consent. Patients with portal vein thrombosis were included if segmental TACE was technically feasible. Patients were included for analysis in this study if they had undergone ≥2 TACE sessions such that sequential arteriograms could be compared. Patient who underwent only a single TACE session without angiographic follow-up were excluded from analysis. Patients who received systemic chemotherapy with sorafenib (Nexavar; Bayer, Leverkusen Germany) and who underwent drug-eluting bead TACE were also excluded from analysis. Previous percutaneous ablation therapy, surgical resection, and intra-arterial therapy using yttrium-90 microspheres prior to TACE therapy were not exclusion criteria, although patients who underwent combination TACE and percutaneous ablation (within two weeks of each other) were excluded.
The diagnosis of HCC was established by percutaneous biopsy or non-invasively based upon the presence of a hepatic mass greater than 2 cm diameter with characteristic imaging findings in the setting of liver cirrhosis and tumor marker elevation (alpha fetoprotein ≥ 200 ng/dL).12 Surgical unresectability was determined by multidisciplinary consensus at tumor conference comprised of medical oncology, transplant surgery, hepatology, and interventional radiology.
Patient demographic and tumor characteristic information is presented in Table 1.
Patient demographics | Tumor characteristics |
---|---|
46 patients (36 men, 10 women) | Tumor focality |
Mean age 59 years (range 37–79 years) | Unifocal (n = 12) |
Liver disease | Multifocal (n = 30) |
Alcoholic (n = 2) | Infiltrative (n = 4) |
Hepatitis C virus (n = 34) | Tumor distribution |
Hepatitis B virus (n = 5) | Unilobar (n = 23) |
Cryptogenic (n = 2) | Bilobar (n = 23) |
NASH (n = 2) | Tumor burden |
Hemochromatosis (n = 1) | <50% (n = 46) |
Child-Pugh score | >50% (n = 0) |
A (n = 19) | Liver segment location of index tumor* |
B (n = 23) | Segment 4 (n = 6) |
C (n = 4) | Segment 5 (n = 4) |
MELD score (mean = 11, range 6–17) | Segment 6 (n = 9) |
Mean AFP 796 ng/dL (range 2–17,231 ng/dL) | Segment 7 (n = 11) |
Pretreatment AFP > 200 ng/dL (n = 11) | Segment 8 (n = 12) |
Diagnosis method | Mean index tumor size* 3.8 × 3.3 cm2 |
Biopsy (n = 32) | Diameter range 0.9–9.4 cm |
Imaging criteria (n = 14) | Previous therapy |
Portal vein thrombosis | RFA (n = 7) |
Lobar (n = 1) | Surgical resection (n = 2) |
Segmental (n = 1) | Yttrium-90 radioembolization (n = 1) |
NASH = non-alcoholic steatohepatitis, MELD = model for end-stage liver disease, AFP = alpha fetoprotein, RFA = radiofrequency ablation,
= among measurable, non-infiltrative tumors (n = 4).
Study cohort.
TACE
TACE procedures were performed in the interventional radiology suite using intravenous moderate sedation. Patients were prepared and draped in standard sterile fashion while supine on the angiographic procedure table, and routine arterial access was generally gained via the right common femoral artery. Initial mapping visceral angiography was then performed using a 5 French visceral catheter (cobra, Sos, or Simmons 1). Subsequent lobar or segmental angiography in the tumor vascular distribution was performed using a 4 French angled glide coated catheter (used in 8/123 procedures) or a coaxially placed 2.3–2.8 French microcatheter (used in 115/123 procedures) after vessel selection with a 0.016, 0.018, or 0.035 inch diameter guidewire. Catheter position was confirmed using DSA with iohexol (Omnipaque 300; Amersham Health, Princeton NJ) injection. After selecting the appropriate catheter position for TACE, chemoembolization was performed with a 1:1, 20 mL volume solution of chemotherapy agents and emulsifying iodized oil (Ethiodol; Savage Laboratories, Melville NY). Chemotherapy regimens followed a “CAM protocol” consisting of cisplatin 100 mg and doxorubicin 50 mg with (n = 119) or without (n = 4) mitomycin C 10 mg. The chemotherapy suspension was injected under direct fluoroscopic observation. Chemotherapy infusion was continued to a substasis angiographic endpoint. Endpoints were retrospectively graded according to the subjective angiographic chemoembolization endpoint (SACE) scale, a scheme designed to assist in reproducibly classifying angiographic TACE endpoints.12 This scale is based on the Thrombolysis In Myocardial Infarction (TIMI) flow grade used in coronary angiography,13 and consists of four levels1: (1) normal antegrade arterial flow and normal or reduced tumor blush, (2) reduced antegrade arterial flow and reduced tumor blush, (3) reduced antegrade arterial flow and no tumor blush, (4) no antegrade arterial flow and no tumor blush. Substasis embolization was defined as SACE levels 1–3, while stasis embolization was defined as SACE level 4. In 21/123 (17%) cases, TACE was concluded by injecting 100–300 μm (n = 3), 300–500 μm (n = 12), or 500–700 μm (n = 6) diameter tris-acryl gelatin microspheres (Embosphere; Biosphere Medical, Rockland MA) mixed with iohexol. Completion arteriography was then performed. Following TACE, all catheters and vascular access devices were removed, and hemostasis was achieved with manual compression (n = 92) or using a vascular closure device (Perclose, Abbott Vascular, Abbott Park IL, n = 15; StarClose, Abbott Vascular, Abbott Park IL, n = 16).
Vascular changes and therapeutic limitation
Sequential hepatic angiographic studies performed on days of treatment were reviewed to assess for abnormalities in appearance of the hepatic vasculature. The hepatic angiogram performed on the day of initial TACE was used to define baseline angiographic appearance, and subsequent arteriograms were compared to angiographic images obtained during most recent prior TACE. Angiographic abnormalities were graded in a binary fashion: present or absent. When present, abnormalities and effect on drug delivery were recorded. Vascular abnormalities were categorized as attenuation or stenosis, slow flow, or occlusion. The vessel location and branch vessel order of the abnormality was also recorded. Vessel order was defined using the following classification: proper hepatic artery = first order vessel, right or left hepatic artery = second order vessel, segmental artery = third order vessel. Additional levels of vessel ramification were duly noted by higher vessel order numbers. Limitation of therapy was defined as inability to administer sufficient chemoembolic material to the same or new tumor vascular distribution (based on fluoroscopic tumor iodized oil uptake) due to flow limitation secondary to hepatic arterial changes from prior TACE.
Statistical analysis
Statistical analyses were implemented in SPSS Version 17 (SPSS Inc., Chicago, IL).
Results
TACE
The 46 patients in the study cohort underwent 123 (mean 2.7, range 2–5) TACE procedures. Lobar (n = 34), segmental (n = 88), or superselective (via direct tumor feeding vessel, n = 1) TACE was successfully performed to substasis (SACE levels 1–3) angiographic endpoints in all cases. Among cases in which administered chemotherapeutic volume was recorded (n = 40, 33%), mean 58% (range 10–100%) of total available chemotherapeutic emulsion volume was infused. Angiographic endpoints in this study corresponded to SACE level 1 (n = 2), SACE 2 (n = 41), and SACE 3 (n = 75). In five cases, post-embolization arteriography was not performed. Three (3/123, 2.4%) procedure-related complications, classified as minor according to the Society of Interventional Radiology Standards of Practice Committee classification of complications,14 included one case of post-embolization syndrome requiring lengthening of hospital admission and two cases of post-procedure transient acute renal failure.
Vascular changes and therapeutic limitation
An abnormal angiographic appearance was identified in 21/46 (38%) patients and in 23/123 (19%) procedures, with first appearance after mean 1.5 (range 1–3) TACE sessions and mean 176 (range 27–509) days after initial TACE. Abnormalities included new vessel attenuation or stenosis (n = 10, 43%) (Fig. 1), slow flow (n = 2, 9%), and new vascular occlusions (n = 11, 48%) (Fig. 2). Abnormalites occurred in 2 s order vessel, seven third order vessels, twelve fourth order vessels, and two fifth order vessels. Mean vessel order of the vascular change was 3.6, corresponding to a segmental or subsegmental vessel. There was no statistical association between TACE procedure number (Fisher Exact test, P = 0.605), lobar or segmental selectively (Fisher Exact test, P = 0.188), use of particle embolic agents (Fisher Exact test, P = 0.224), or SACE level (Fisher Exact test, P = 0.812) and the presence of arterial vascular change. Analysis of the relationship between chemotherapy dose administered and presence of vascular change was precluded because administered drug dose was not recorded in 83/123 (67%) TACE procedures.
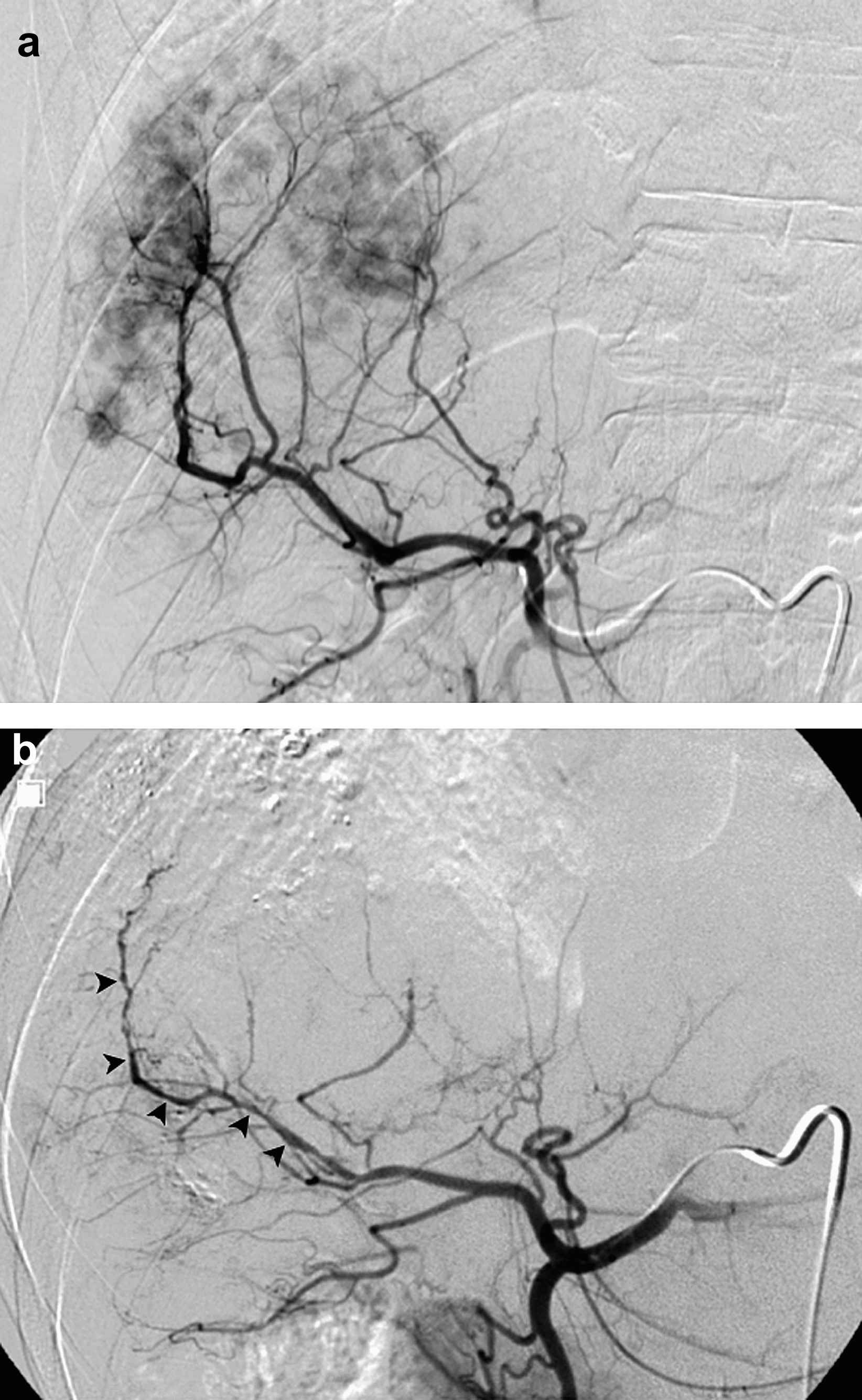
51-year-old man with hepatitis C virus liver disease and biopsy proven multifocal recurrent HCC after radiofrequency ablation. Arteriogram (a) during TACE demonstrates normal branching and caliber of right hepatic arteries. Arteriogram (b) performed during repeat TACE 59 days later shows new multifocal stenoses (black arrowheads) of right hepatic segmental branch vessel.

48-year-old woman with hepatitis C virus liver disease and biopsy proven unifocal HCC. Arteriogram (a) during TACE reveals hypervascular tumor supplied by segment 6 artery (arrowhead), from which selective TACE performed. Arteriogram (b) during TACE performed 279 days later for treatment of left hepatic lobe tumor demonstrates new occlusion of segment 6 vessel (arrowhead).
In 16/23 (70%) cases where vascular changes were present, repeat TACE was performed to the liver lobe with the hepatic arterial injury. In 7/23 (30%) cases, TACE was performed to the contralateral liver lobe for treatment of bilobar tumor. TACE was successfully performed in 16/16 (100%) cases where vascular changes were present and TACE was repeated to the same liver lobe. In 6/16 (38%) cases, there was no therapy limiting flow impediment from the existing vascular abnormality (Fig. 3). In 10/16 (62%) cases, TACE was performed via other ipsilateral hepatic arterial tumor feeding branch vessels. Analysis of differences in injectable chemotherapy volume between cases with and without vascular change was not possible due to lack of chemotherapy dose data.
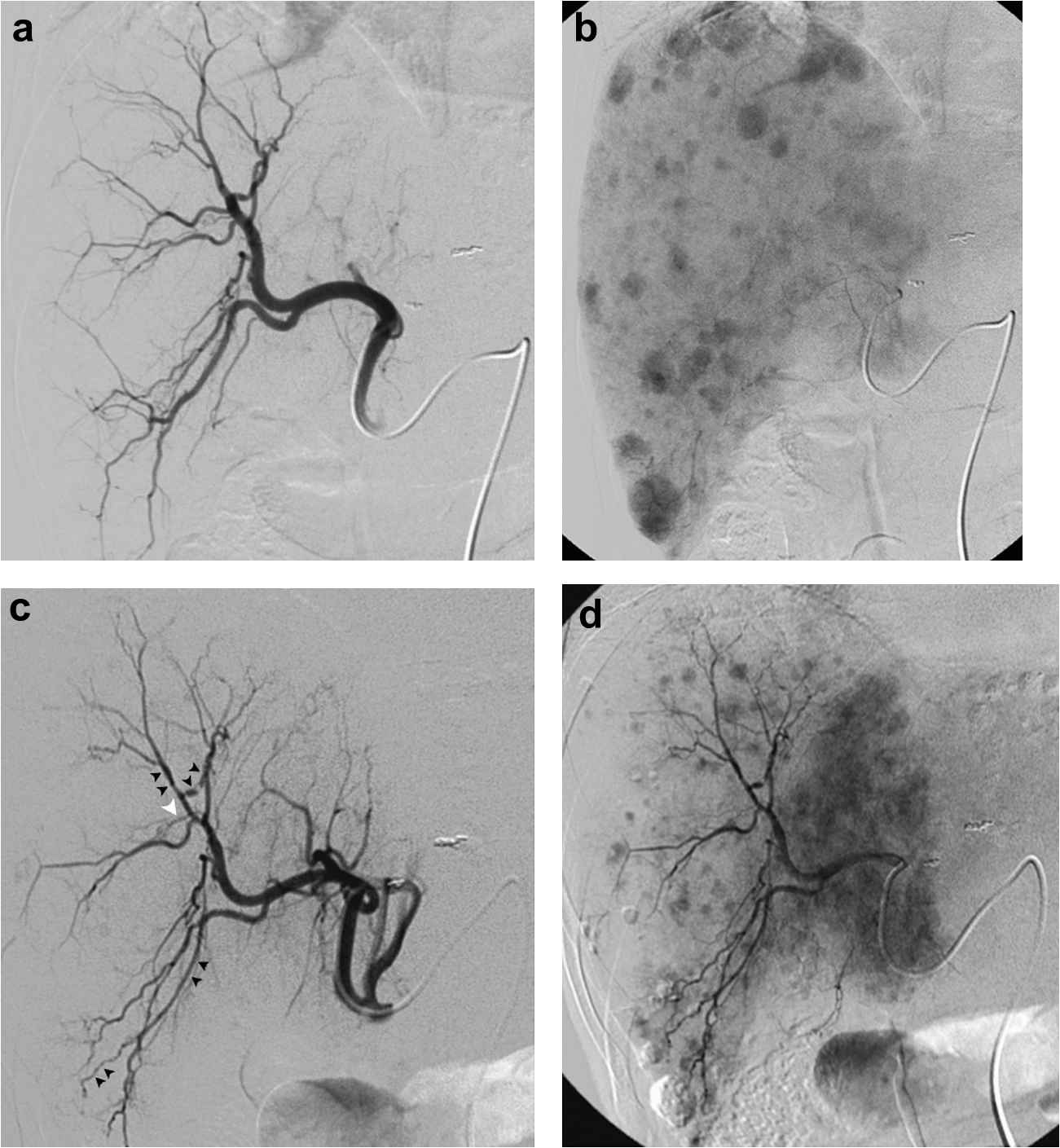
61-year-old man with hepatitis C virus liver disease and biopsy proven multifocal bilobar HCC. Arteriogram (a) during TACE shows normal ramification and quality of right hepatic arteries. Delayed imaging (b) shows multifocal tumor blush, and lobar iodized oil TACE uneventfully performed. Arteriogram (c) during repeat TACE 109 days later shows new attenuation (black arrowheads) and occlusion (white arrowhead) of right hepatic arterial branches. Repeat right hepatic lobe TACE performed without significant flow impediment for treatment of new multifocal tumor blush (d).
Discussion
Hepatocellular carcinoma (HCC) is a significant cause of morbidity and mortality in the United States.15–17 Liver tumors accounted for over 18,000 deaths in 2008,16 and the incidence of HCC is rising.15 Surgical resection represents a curative treatment for HCC. However, only a minority of patients with this malignancy are candidates for hepatectomy due to advanced multifocal disease, poor hepatic reserve, or significant extrahepatic tumor burden.15,16 Hepatic transplantation is another surgical treatment option for HCC, but is significantly limited by lack of donor organs. Due to the limitations of these treatment methods, TACE plays a large role in the treatment of patients with HCC.18 When performed using an iodized oil and polychemotherapy regimen, TACE shows excellent disease control in surgically unresectable HCC.19
Typically, the beneficial clinical effects of TACE are related to a combination of the cytotoxic effects of chemotherapy agents on cancer cells and the hypoxic effects of transient vessel embolization induced by iodized oil. Chemotherapy agents traditionally used in chemoembolization performed in the United States include cisplatin, doxorubicin, and mitomycin C emulsified in iodinated oily contrast medium.5 Chemotherapeutic drugs such as those used in chemoembolization are known to be hazardous, and have recognized carcinogenic, mutagenic, and possibly teratogenic effects.20 Due to the toxic nature of these substances, chemotherapy agents may result in injury to the vascular endothelium, resulting in hepatic arteriographic changes, including attenuation, stenosis, slow flow, and occlusion.7,21 Stasis of chemotherapy and prolonged contact of cytotoxic agents with the vascular endothelium has been suggested to represent the major contributing factor for arterial injury,22 a generally undesirable side effect that by and large detrimental and disadvantageous for at least two reasons. First, arterial occlusion due to chemotherapy related vessel injury has the potential to preclude liver vessel access for future therapy, and advocates of alternative intra-arterial therapies, such as bland particle embolization and yttrium-90 microsphere radioembolization, may identify arterial injury and limitation of future therapy as a significant disadvantage of TACE. Second, prolonged arterial flow limitation or occlusion to a level beyond therapeutic benefit may induce tumor angiogenesis and recurrence.23,24 To this end, minimization of widespread chemotherapy induced vessel damage is vital, and embolization to a substasis angiographic endpoint may be beneficial by allowing earlier washout of toxic chemotherapy agents from large feeding hepatic arteries and arterioles.
The incidence of hepatic arterial damage resulting from TACE has been reported using several different chemoembolic regimens. Belli et al. described new hepatic arterial stenoses and occlusions in 7/117 (6%) patients with HCC (61/117, 52%) and metastatic liver disease (56/117, 48%) treated with 1–4 TACE sessions using epirubicin and mitomycin C emulsified in lipiodized oil followed by gelatin sponge injection.9 Vascular changes were seen after one TACE session in five patients and after two TACE sessions in two patients, and the authors found no correlation between drug or gelatin sponge dose and the presence of vascular injury.9 Geschwind et al. reported on arterial patency in 160 patients with primary or secondary liver cancer that underwent TACE using cisplatin, doxorubicin, and mitomycin C emulsified in ethiodized oil either1 mixed in a slurry with polyvinyl alcohol (PVA) particles (36/160, 23%),2 followed by PVA particles (91/160, 57%), or3 followed by gelfoam pledgets (33/160, 20%).10 In all patients, TACE was continued until the entire prescribed chemotherapy dose was administered or until near stasis (90% flow reduction) in tumor feeding arteries was achieved.10 In this study, administration of PVA embolic agent as a slurry with the chemotherapy emulsion reduced the injectable volume of chemotherapy (55% in group 1 versus 75% and 81% in groups 2 and 3, respectively) and significantly reduced arterial patency as compared to administration of embolic agent after chemotherapy emulsion (56% in group 1 versus 74% and 81% in groups 2 and 3, respectively).10 Maeda et al. reported hepatic arterial damage in 37/231 (16%) treated subsegmental arteries and 16/33 (48%) patients with HCC treated with TACE consisting of epirubicin, lipiodized oil, and gelatin sponge administered to an angiographic endpoint of flow cessation.11 The authors found that accumulated dose of epirubicin represented a significant predictor of hepatic arterial damage.11
We undertook the current study to assess the arteriographic and therapeutic consequences of iodized oil TACE performed to a substasis angiographic endpoint. Our study, which showed 19% incidence of arteriographic changes, corroborated the findings Maeda et al., where a 16% rate of arterial injury was seen. The rate of arterial patency in our study was improved as compared to results reported by Geschwind et al.,10 presumably due to the fact that TACE was performed to a substasis angiographic endpoint in which antegrade arterial flow was reduced but maintained, and because particulate embolic agents were not mixed into the chemoembolic slurry. This hypothetically allows for more rapid washout of toxic chemotherapeutic agents. Interestingly, we found no association between TACE procedure number, lobar or segmental selectively, use of particle embolic agents, or SACE level and the presence of arterial vascular change. Analysis of the injected volume of chemotherapy agent and the presence of vascular change was not possible herein due to lack of data, and the relationship between accumulated chemotherapy dose and the presence of arterial injury in the setting of substasis TACE requires further investigation.
Recently, Erinjeri et al.22 described the arterial patency after repeated bland particle embolization for treatment of HCC. In this study, 272 embolizations were performed in 43 patients generally using 50 μm PVA particles or 40–120 μm followed by 300–500 μm tris-acryl gelatin microspheres without chemotherapy agents or iodized oil.22 In this cohort, embolization was performed to a stasis angiographic endpoint.22 36/43 (83%) patients showed no change in hepatic arteriographic appearance, and 7/43 (16%) patients exhibited occlusion of a fourth (n = 1) or fifth (n = 6) order vessel after an average of five embolizations over 34 months.22 Based on the results of our study, the per patient and per procedure incidence of vascular changes appears to be greater in TACE as compared to bland particle embolization. However, the arteriographic changes did not result in significant therapeutic consequence, as TACE was technically successful in 100% of cases where vascular changes were present and TACE was repeated to the same liver lobe in this series. These results suggest that the arterial damage induced by CAM protocol iodized oil TACE performed to a substasis level does not preclude technically successful repeat TACE therapy. Furthermore, when present, vascular changes were generally located at the segmental or subsegmental arterial level, corresponding to a point in the hepatic arterial tree where flow limitation may not be as important for subsequent TACE. Given the results of this study and the outcome benefits seen by the addition of chemotherapy to intra-arterial HCC treatment regimens,1,25 the authors advocate use of TACE rather than bland embolization in HCC therapy.
There were several important limitations to this study. First, this study is retrospective in nature, and is subject to the inherent weaknesses of non-prospective studies. Second, arterial injuries were diagnosed on the basis of angiographic change, and no histopathologic confirmation was available. Third, the timeline of development of vascular changes herein is subject to uncertainty because repeat TACE was required in order to obtain angiographic assessment of the hepatic arterial vasculature. As such, early occurring vascular changes may not have been identified until weeks or months after development until repeat hepatic angiography was performed. Fourth, we were unable to perform an analysis of the relationship between chemotherapy dose administered and presence of vascular change because administered drug dose was not recorded in most TACE procedures. Fifth, the effect of drug-eluting bead TACE on the hepatic vasculature was not evaluated in this investigation. Finally, we did not correlate the angiographic findings with imaging and clinical outcomes in this study to assess the impact of vascular change on TACE response.
In summary, iodized oil TACE performed to a substasis angiographic endpoint was associated with a non-trivial rate of hepatic arterial injury changes in this study. However, these angiographic changes did not result in limitation of therapy during subsequent TACE sessions. Despite the limitations of this investigation, these findings support the need for a larger prospective trial to assess the effects of chemotherapy on the hepatic vasculature and to optimize TACE regimens to minimize arterial injury.
Financial support, disclosures, and conflicts of interest
None.
Acknowledgments
None.
References
Cite this article
TY - JOUR AU - Ron C. Gaba AU - Tamara R. Brodsky AU - M. Grace Knuttinen AU - Benedictta O. Omene AU - Charles A. Owens AU - James T. Bui PY - 2011 DA - 2011/09/07 TI - Hepatic arterial changes following iodized oil chemoembolization of hepatocellular carcinoma: Incidence and technical consequence JO - Artery Research SP - 21 EP - 27 VL - 6 IS - 1 SN - 1876-4401 UR - https://doi.org/10.1016/j.artres.2011.08.002 DO - 10.1016/j.artres.2011.08.002 ID - Gaba2011 ER -